BBYCT-135 Solved Assignment
-
a) Define the following terms :
i) Quiescent center
ii) Mangroves
iii) Adventive embryos
iv) Tyloses
v) Dichogamy
vi) Apomixis
vii) Cross pollination
viii) Osteosclereids
ix) Phelloderm
x) Heterophylly -
a) Enlist the major characteristic features of halophytes.
b) List various types of simple and complex tissues. Describe complex tissues with suitable diagrams. -
Describe the structure of embryo sac with the help of a well labelled diagram.
-
a) List various types of ovules and describe them with outline diagrams.
b) Define apomixis and list its types. Discuss various causes of apomixis and its importance. -
Enlist the categories of xerophytes. Describe the morphological and anatomical adaptations found in xerophytes.
-
a) What is parthenocarpy. Describe various types of parthenocarpy found in plants.
b) Explain incompatibility and describe its types and significance. -
With the help of well labeled diagram describe the ABC Model of Flower Organization.
-
Describe syngamy and triple fusion in angiosperms with the help of a well labelled diagram. Enlist the major functions of endosperm in plants.
-
Differentiate between:
i) Porous and non-porous wood
ii) Dicot stem and monocot stem
iii) Open and closed style
iv) Heartwood and sapwood
v) Glandular and non-glandular trichomes -
Write short notes on:
i) Aerial roots
ii) Rhizomes
iii) Cambial variant in stems
iv) Dendrochronology
Answer:
Question:-1(a)
Define the following terms:
i) Quiescent center
ii) Mangroves
iii) Adventive embryos
iv) Tyloses
v) Dichogamy
vi) Apomixis
vii) Cross pollination
viii) Osteosclereids
ix) Phelloderm
x) Heterophylly
ii) Mangroves
iii) Adventive embryos
iv) Tyloses
v) Dichogamy
vi) Apomixis
vii) Cross pollination
viii) Osteosclereids
ix) Phelloderm
x) Heterophylly
Answer:
Definitions of Terms:
-
Quiescent Center
A region of slowly dividing cells located in the root apical meristem of plants. It acts as a reservoir of stem cells, maintaining meristem activity and regenerating damaged root tissues. Found in the root tip, it ensures long-term growth and repair. -
Mangroves
Salt-tolerant trees or shrubs (e.g., Rhizophora, Avicennia) inhabiting tropical/subtropical coastal zones. Adapted with pneumatophores (aerial roots), viviparous seeds, and salt-excreting leaves. Vital for coastal protection and biodiversity. -
Adventive Embryos
Embryos formed asexually from somatic cells (e.g., nucellus, integuments) rather than fertilization. A form of apomixis, seen in plants like citrus, producing multiple embryos per seed (polyembryony). -
Tyloses
Balloon-like outgrowths from xylem parenchyma cells into adjacent vessels or tracheids. They block water transport during stress or infection, contributing to heartwood formation and pathogen resistance in woody plants. -
Dichogamy
Temporal separation of male (anther) and female (stigma) maturation in a flower to prevent self-pollination. Types:- Protandry: Anthers release pollen before stigma is receptive (e.g., sunflowers).
- Protogyny: Stigma matures first (e.g., avocados).
-
Apomixis
Asexual seed production without fertilization. Offspring are maternal clones. Common in dandelions and grasses, via mechanisms like adventive embryony or gametophytic apomixis. -
Cross Pollination
Transfer of pollen from the anther of one plant to the stigma of another. Enhances genetic diversity, mediated by wind, insects, or birds. Promoted by mechanisms like dichogamy or self-incompatibility. -
Osteosclereids
Bone-shaped sclereids with thickened cell walls, providing structural support. Found in seed coats (e.g., peas) and leaves, contributing to tissue rigidity and defense. -
Phelloderm
The innermost layer of the periderm (bark), produced inward by the cork cambium (phellogen). Consists of living parenchyma cells, aiding in storage and secondary growth in woody stems. -
Heterophylly
Occurrence of distinct leaf shapes on the same plant, driven by environmental factors (e.g., aquatic vs. terrestrial leaves in water crowfoot) or developmental stages (e.g., juvenile vs. mature eucalyptus leaves). Enhances ecological adaptability.
Question:-2(a)
Enlist the major characteristic features of halophytes.
Answer:
1. Definition of Halophytes
Halophytes are specialized plants that thrive in saline environments, where the soil or water contains high concentrations of salt. The term "halophyte" is derived from the Greek words "halas," meaning salt, and "phyton," meaning plant. These plants have developed unique adaptations that allow them to survive and flourish in conditions that would be detrimental to most other plant species, known as glycophytes. Halophytes can be found in various habitats, including coastal regions, salt marshes, mangrove swamps, and saline deserts.
2. Classification of Halophytes
Halophytes can be classified based on several criteria, including their habitat and the degree of salinity they can tolerate:
-
By Habitat:
- Aqua-halines: Aquatic halophytes that can be further divided into emerged halophytes (partially above water) and hydro-halophytes (submerged).
- Terrestro-halines: Terrestrial halophytes categorized into hygro-halophytes (wetland species), mesohalophytes (moderate salinity), and xero-halophytes (dry land).
- Aero-halines: Epiphytic or aerophytic species that grow on other plants.
-
By Salinity Tolerance:
- Oligo-halophytes: Tolerate low salinity (0.01 to 0.1% NaCl).
- Meso-halophytes: Tolerate moderate salinity (0.1 to 1% NaCl).
- Euhalophytes: Thrive in high salinity (>1% NaCl).
This classification helps in understanding the ecological roles and adaptations of different halophyte species.
3. Physiological Adaptations
Halophytes possess a range of physiological adaptations that enable them to cope with high salinity:
-
Salt Excretion Mechanisms: Many halophytes have specialized salt glands that excrete excess salt from their tissues, allowing them to maintain a lower internal concentration of salts. This process helps prevent toxic buildup within the plant.
-
Osmotic Adjustment: Halophytes often accumulate compatible solutes such as proline and glycine betaine, which help maintain osmotic balance without disrupting cellular functions.
-
Ion Compartmentalization: These plants can compartmentalize excess sodium ions into vacuoles, effectively isolating them from metabolic processes.
These adaptations ensure that halophytes can not only survive but also grow optimally in saline conditions.
4. Morphological Characteristics
The morphology of halophytes is often distinct and adapted for saline environments:
-
Leaf Structure: Halophytic leaves are typically thick, succulent, and may have a glassy appearance due to the presence of salt crystals on their surfaces. Some species have reduced leaf sizes or may even be leafless (aphyllous) to minimize water loss.
-
Root Systems: Halophytes usually develop extensive root systems that allow for better water uptake from deeper soil layers. Some species have specialized roots known as prop roots that provide stability in unstable substrates like mudflats.
-
Growth Form: Many halophytes exhibit a bushy or sprawling growth form to maximize light capture and minimize water loss through transpiration.
These morphological traits enhance their survival in challenging environments.
5. Ecological Importance
Halophytes play a crucial role in their ecosystems:
-
Habitat Formation: Mangroves and salt marshes formed by halophytic vegetation provide critical habitats for various marine and terrestrial organisms, including fish, birds, and invertebrates.
-
Soil Stabilization: The extensive root systems of halophytes help stabilize soils in coastal areas, preventing erosion and maintaining ecosystem integrity.
-
Nutrient Cycling: Halophytes contribute to nutrient cycling within their ecosystems by accumulating salts and organic matter, which enriches the soil when they die and decompose.
Their ecological functions are vital for maintaining biodiversity and healthy ecosystems in saline environments.
6. Agricultural Applications
Research into halophytes has significant implications for agriculture, particularly in areas affected by soil salinity:
-
Saline Agriculture: Halophytes can be cultivated as crops in saline soils where traditional crops fail. They can produce valuable products such as biofuels, fodder, and food while utilizing otherwise unproductive land.
-
Phytoremediation: Some halophytic species are being studied for their ability to remediate contaminated soils by absorbing heavy metals and other pollutants alongside excess salts.
-
Drought Resistance: The mechanisms developed by halophytes to cope with salinity may also confer drought resistance, making them valuable for future agricultural practices in changing climates.
These applications highlight the potential of halophytes in sustainable agriculture and environmental management.
Conclusion
Halophytes represent a remarkable group of plants uniquely adapted to thrive in saline environments. Their classification, physiological adaptations, morphological characteristics, ecological importance, and agricultural applications underscore their significance in both natural ecosystems and human endeavors. As research continues to explore the potential of these salt-tolerant plants, they may offer innovative solutions to some of the pressing challenges posed by soil salinity and climate change.
Question:-2(b)
List various types of simple and complex tissues. Describe complex tissues with suitable diagrams.
Answer:
Types of Simple and Complex Tissues
Tissues are groups of cells with similar structure and function that work together to perform specific roles in an organism. They are broadly classified into simple tissues (composed of a single cell type) and complex tissues (composed of multiple cell types). Below is a detailed list and description, focusing on plant tissues as the context suggests a biological focus, followed by descriptions and diagrams of complex tissues.
Simple Tissues
Simple tissues consist of one type of cell and are primarily found in plants. They perform functions like storage, photosynthesis, or protection.
-
Parenchyma
- Characteristics: Living cells with thin cell walls, large central vacuole, and intercellular spaces.
- Function: Photosynthesis, storage (of starch, water, etc.), and tissue repair.
- Location: Found in cortex, pith, mesophyll of leaves, and fruit pulp.
-
Collenchyma
- Characteristics: Living cells with unevenly thickened cell walls (rich in cellulose and pectin).
- Function: Provides mechanical support and flexibility to growing parts.
- Location: Below the epidermis in stems and leaf petioles.
-
Sclerenchyma
- Characteristics: Dead cells with thick, lignified cell walls. Two types: fibers (long, narrow) and sclereids (short, irregular).
- Function: Provides rigidity and strength.
- Location: In stems, seed coats, and nutshells.
Complex Tissues
Complex tissues are composed of multiple cell types working together to perform specialized functions. In plants, the two main complex tissues are xylem and phloem, which form the vascular system responsible for transport.
Detailed Description of Complex Tissues
1. Xylem
- Composition: Xylem is a complex tissue responsible for conducting water and minerals from roots to other parts of the plant. It consists of:
- Tracheids: Long, narrow, dead cells with tapered ends and pitted walls. They conduct water and provide structural support.
- Vessels (Tracheae): Wider, tube-like dead cells with perforated end walls, allowing efficient water transport. Found mainly in angiosperms.
- Xylem Parenchyma: Living cells that store nutrients and assist in lateral transport.
- Xylem Fibers: Dead, lignified cells that provide mechanical strength.
- Function:
- Transports water and dissolved minerals unidirectionally (from roots to leaves).
- Provides mechanical support due to lignified cells.
- Location: Found in vascular bundles of roots, stems, and leaves.
- Types:
- Primary Xylem: Formed during initial growth (protoxylem and metaxylem).
- Secondary Xylem: Formed in woody plants during secondary growth (e.g., wood).
Diagram of Xylem:
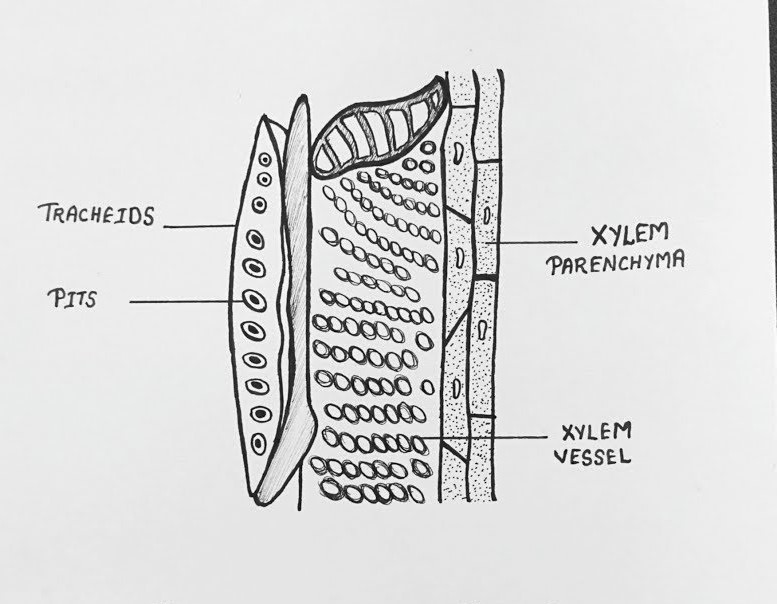
2. Phloem
- Composition: Phloem is a complex tissue responsible for transporting organic nutrients (e.g., sugars) produced during photosynthesis. It consists of:
- Sieve Tubes: Living cells with perforated end walls (sieve plates) that form tubes for nutrient transport. They lack nuclei at maturity.
- Companion Cells: Living cells with dense cytoplasm and nuclei, controlling the activities of sieve tubes.
- Phloem Parenchyma: Living cells for storage and lateral transport (absent in some monocots).
- Phloem Fibers: Dead, lignified cells providing structural support.
- Function:
- Transports organic compounds (e.g., sucrose) bidirectionally (from leaves to roots, fruits, or storage organs).
- Supports nutrient distribution in the plant.
- Location: Found in vascular bundles alongside xylem.
- Types:
- Primary Phloem: Formed during initial growth (protophloem and metaphloem).
- Secondary Phloem: Formed in woody plants during secondary growth (e.g., bark).
Diagram of Phloem:
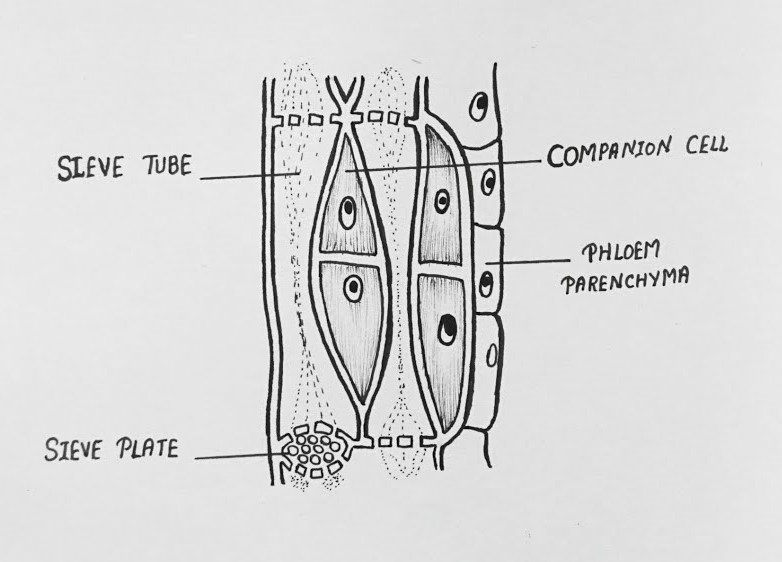
Key Points
- Simple tissues (parenchyma, collenchyma, sclerenchyma) are uniform in structure and perform basic functions like storage, support, and photosynthesis.
- Complex tissues (xylem, phloem) are specialized for transport and contain multiple cell types, making them critical for plant survival.
- Diagrams of complex tissues typically show transverse sections of vascular bundles, where xylem and phloem are arranged together (e.g., in dicot stems, xylem is internal, and phloem is external).
Question:-3
Describe the structure of embryo sac with the help of a well labelled diagram.
Answer:
Structure of Embryo Sac
The embryo sac, also known as the female gametophyte, is the structure within the ovule of flowering plants (angiosperms) where fertilization occurs. It is formed through the process of megasporogenesis and megagametogenesis and is typically a 7-celled, 8-nucleate structure in the most common type, known as the Polygonum type. Below is a detailed description of its structure, followed by a well-labeled diagram.
Description of Embryo Sac Structure
The embryo sac is housed within the ovule, which is located inside the ovary of the flower. It develops from a single functional megaspore that undergoes mitotic divisions to form the mature embryo sac. The key components of the embryo sac are:
-
Egg Apparatus (at the micropylar end):
- Egg Cell: A large, haploid cell that fuses with one sperm nucleus during fertilization to form the diploid zygote, which develops into the embryo.
- Synergids (two): Flanking the egg cell, these haploid cells have finger-like projections called filiform apparatus at the micropylar end. They assist in guiding the pollen tube to the egg cell and may degenerate after fertilization.
-
Central Cell:
- The largest cell in the embryo sac, it contains two polar nuclei (haploid) that fuse with the second sperm nucleus during double fertilization to form the triploid primary endosperm nucleus. This nucleus develops into the endosperm, which nourishes the embryo.
- The central cell is filled with a large vacuole and has thin cytoplasm.
-
Antipodal Cells (at the chalazal end):
- Typically three haploid cells located opposite the micropylar end.
- Their function is not fully understood, but they may play a role in nutrient transfer to the embryo sac or degenerate after fertilization.
-
Micropylar and Chalazal Ends:
- The micropylar end is the opening through which the pollen tube enters the embryo sac.
- The chalazal end is opposite, near the ovule’s vascular supply.
-
Nuclei and Ploidy:
- The embryo sac is 8-nucleate: 1 egg nucleus, 2 synergid nuclei, 2 polar nuclei, and 3 antipodal nuclei.
- All nuclei are haploid except the primary endosperm nucleus (triploid) formed after fertilization.
Formation of Embryo Sac (Brief Overview)
- A megaspore mother cell in the ovule undergoes meiosis to produce four megaspores.
- Three megaspores degenerate, leaving one functional megaspore.
- The functional megaspore undergoes three mitotic divisions without cytokinesis, forming an 8-nucleate structure.
- Cytokinesis then organizes the nuclei into 7 cells: 1 egg cell, 2 synergids, 1 central cell (with 2 polar nuclei), and 3 antipodal cells.
Well-Labelled Diagram of Embryo Sac
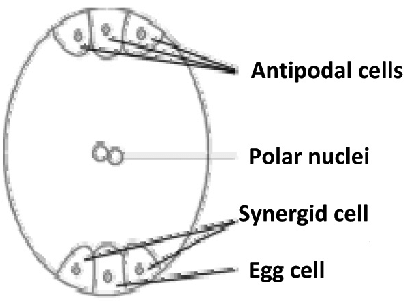
- Micropylar End: Where the pollen tube enters.
- Egg Cell: Large cell for zygote formation.
- Synergids: Cells with filiform apparatus guiding pollen tube.
- Central Cell: Contains two polar nuclei for endosperm formation.
- Antipodal Cells: Three cells at the chalazal end.
- Chalazal End: Opposite the micropylar end, near nutrient supply.
- The embryo sac is pear-shaped or oval, with the micropylar end narrower and the chalazal end broader.
- The egg apparatus is tightly packed at the micropylar end, with synergids showing filiform apparatus (protrusions).
- The central cell occupies the bulk of the embryo sac, with two prominent polar nuclei in the center.
- Antipodal cells are smaller and clustered at the chalazal end.
- The embryo sac is surrounded by the nucellus and integuments of the ovule.
- The Polygonum type (7-celled, 8-nucleate) is the most common embryo sac structure, but variations like the Oenothera type (4-celled) or Allium type exist.
- The embryo sac is critical for double fertilization, a unique feature of angiosperms, where one sperm fertilizes the egg (forming the zygote) and the other fuses with the polar nuclei (forming the endosperm).
Question:-4(a)
List various types of ovules and describe them with outline diagrams.
Answer:
Types of Ovules and Their Descriptions with Outline Diagrams
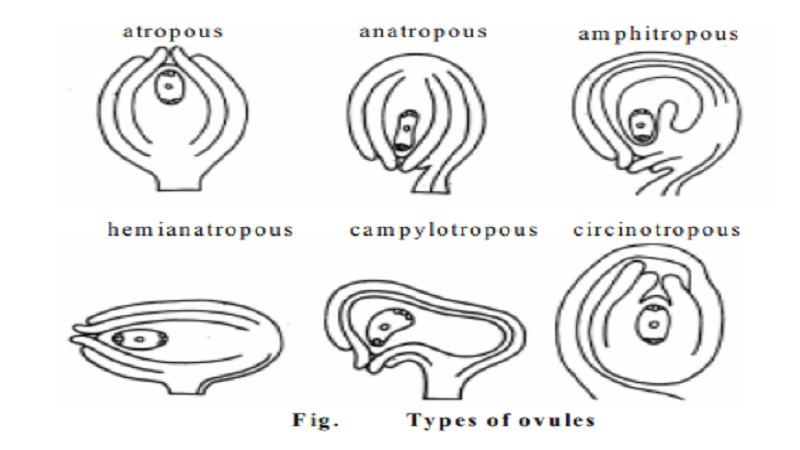
In flowering plants (angiosperms), the ovule is a structure within the ovary that contains the female gametophyte (embryo sac) and develops into a seed after fertilization. Ovules are classified based on the orientation of the ovule body relative to the funicle (stalk) and the position of the micropyle (opening for pollen tube entry) relative to the chalaza (region opposite the micropyle).
Types of Ovules
There are six main types of ovules based on their structure and orientation:
- Orthotropous (Atropous)
- Anatropous
- Hemitropous (Hemianatropous)
- Campylotropous
- Amphitropous
- Circinotropous
Descriptions and Outline Diagrams
1. Orthotropous (Atropous) Ovule
- Description:
- The simplest and most primitive type of ovule.
- The ovule is erect, with the micropyle, funicle, and chalaza aligned in a straight line.
- The funicle is attached at the base, and the micropyle faces upward.
- Found in plants like Polygonaceae (e.g., buckwheat) and Piperaceae (e.g., pepper).
- Function: Provides a straightforward path for pollen tube entry but is less common in advanced angiosperms.
2. Anatropous Ovule
- Description:
- The most common type of ovule in angiosperms (e.g., found in Asteraceae, Fabaceae).
- The ovule is inverted (turned 180°), so the micropyle is close to the funicle and faces downward.
- The body of the ovule is fused to the funicle along its length, forming a raphe (a ridge of fused tissue).
- The chalaza is at the opposite end from the micropyle.
- Function: The inverted position facilitates pollen tube entry near the placenta.
3. Hemitropous (Hemianatropous) Ovule
- Description:
- The ovule is intermediate between orthotropous and anatropous, bent at a 90° angle.
- The micropyle and chalaza are at right angles to the funicle.
- The ovule body is partially inverted, with the funicle attached at the middle.
- Found in plants like Ranunculaceae (e.g., buttercup).
- Function: Represents a transitional form, balancing erect and inverted orientations.
4. Campylotropous Ovule
- Description:
- The ovule is curved, but not fully inverted, forming a C-shaped structure.
- The micropyle is close to the chalaza, and the funicle is attached at the base or side.
- The curvature causes the embryo sac to bend, but the ovule does not fuse with the funicle (no raphe).
- Found in plants like Brassicaceae (e.g., mustard) and Caryophyllaceae (e.g., carnation).
- Function: The curved shape optimizes space within the ovary and aids pollen tube entry.
5. Amphitropous Ovule
- Description:
- The ovule is curved more than campylotropous, with the body bent so the micropyle and chalaza are near each other.
- The funicle is attached near the middle, and the embryo sac is strongly curved (horseshoe-shaped).
- Found in plants like Malvaceae (e.g., cotton) and Chenopodiaceae (e.g., spinach).
- Function: The extreme curvature maximizes space efficiency and aligns the micropyle for fertilization.
6. Circinotropous Ovule
- Description:
- A rare type where the ovule is coiled or twisted in a 360° loop.
- The funicle is long and wraps around the ovule, causing the micropyle to face in an unpredictable direction.
- Found in plants like Cactaceae (e.g., cactus) and Plumbaginaceae (e.g., sea lavender).
- Function: The coiling may protect the ovule or align it uniquely within the ovary.
Question:-4(b)
Define apomixis and list its types. Discuss various causes of apomixis and its importance.
Answer:
Definition of Apomixis
Apomixis is a form of asexual reproduction in plants where seeds are produced without fertilization, resulting in offspring that are genetically identical to the parent plant. In apomixis, the embryo develops from an unfertilized egg cell or other somatic cells within the ovule, bypassing meiosis and gamete fusion. This process mimics sexual reproduction by producing seeds, but it maintains the genetic makeup of the mother plant.
Types of Apomixis
Apomixis is classified into three main types based on the mechanism of embryo formation and the cells involved:
-
Diplospory:
- The embryo sac develops from the megaspore mother cell (MMC) or a cell derived from it without undergoing meiosis.
- The MMC divides mitotically to form an unreduced (diploid) embryo sac, and the egg cell develops into an embryo parthenogenetically (without fertilization).
- Example: Found in plants like Taraxacum (dandelion) and Allium (onion).
- Subtypes:
- Meiotic diplospory: Meiosis starts but is incomplete, producing unreduced cells.
- Mitotic diplospory: Meiosis is entirely skipped.
-
Apospory:
- The embryo sac forms from a somatic cell (usually in the nucellus or integuments) rather than the MMC.
- This somatic cell divides mitotically to produce an unreduced embryo sac, and the egg cell develops into an embryo without fertilization.
- Example: Found in Hieracium (hawkweed) and Pennisetum (fountain grass).
- Apospory often coexists with sexual reproduction in the same plant, producing both apomictic and sexual seeds.
-
Adventitious Embryony (Nucellar Embryony):
- The embryo develops directly from somatic cells of the nucellus or integuments, bypassing the formation of an embryo sac.
- Multiple embryos (polyembryony) may form in a single ovule, often alongside a sexually produced embryo.
- Example: Found in Citrus (oranges, lemons) and Mangifera (mango).
Causes of Apomixis
Apomixis is a complex trait influenced by genetic, environmental, and physiological factors. The causes can be summarized as follows:
-
Genetic Factors:
- Apomixis is often controlled by specific genes or gene complexes, such as APOMIXIS-REGULATING loci.
- Mutations or alterations in genes regulating meiosis, gametophyte development, or embryo formation can lead to apomixis.
- Polyploidy (having multiple sets of chromosomes) is strongly associated with apomixis, as it stabilizes unreduced gametes and supports asexual seed formation.
- Hybridization between species can disrupt normal sexual reproduction, triggering apomictic pathways.
-
Epigenetic Regulation:
- Changes in gene expression due to DNA methylation, histone modification, or small RNAs can suppress meiosis or fertilization, promoting apomixis.
- Epigenetic reprogramming in the ovule may favor mitotic divisions over meiotic ones.
-
Hormonal Imbalances:
- Alterations in plant hormones (e.g., auxins, cytokinins) can induce somatic cells to form embryos or embryo sacs.
- Stress-induced hormonal changes may trigger apomictic development as a survival mechanism.
-
Environmental Stress:
- Factors like temperature extremes, drought, or nutrient deficiency can disrupt sexual reproduction, favoring apomixis as an adaptive response.
- Apomixis allows plants to reproduce under conditions where pollinators or suitable mates are scarce.
-
Evolutionary Adaptation:
- Apomixis may arise as an evolutionary strategy to maintain successful genotypes in stable environments or colonize new habitats without relying on sexual reproduction.
- It is more common in polyploid and hybrid plants, which often face reproductive barriers in sexual reproduction.
Importance of Apomixis
Apomixis has significant ecological, agricultural, and evolutionary implications:
-
Agricultural Applications:
- Fixation of Hybrid Vigor: Apomixis allows the propagation of hybrid plants with desirable traits (e.g., high yield, disease resistance) without segregation in subsequent generations. This is particularly valuable for crops like maize, rice, and wheat.
- Seed Production: Apomictic plants produce seeds without pollination, reducing dependency on pollinators and ensuring seed set in adverse conditions.
- Cost Efficiency: Farmers can reuse apomictic seeds, reducing the need to purchase new hybrid seeds annually.
- Crop Improvement: Apomixis can be engineered into crops to stabilize elite genotypes, accelerating breeding programs.
-
Ecological Advantages:
- Colonization: Apomictic plants can colonize new or disturbed habitats rapidly due to their ability to reproduce without mates or pollinators.
- Genetic Uniformity: Apomixis maintains successful genotypes in stable environments, ensuring survival of well-adapted plants.
- Reproductive Assurance: In areas with low pollinator activity or isolated populations, apomixis ensures seed production.
-
Evolutionary Significance:
- Apomixis allows plants to bypass the genetic recombination of sexual reproduction, preserving advantageous gene combinations.
- It is often associated with polyploidy and hybridization, contributing to speciation and diversity in certain plant groups (e.g., Asteraceae, Poaceae).
- However, apomixis may limit long-term adaptability due to reduced genetic variation.
-
Research and Biotechnology:
- Understanding apomixis can lead to breakthroughs in plant breeding by enabling the transfer of apomictic traits to sexually reproducing crops.
- Apomixis research helps unravel the genetic and molecular mechanisms of reproduction, providing insights into plant development and evolution.
-
Challenges:
- Apomixis can lead to reduced genetic diversity, making populations vulnerable to environmental changes or diseases.
- In some cases, apomictic weeds (e.g., dandelions) can become invasive due to their prolific seed production.
Summary
- Definition: Apomixis is asexual seed production resulting in genetically identical offspring.
- Types: Diplospory, apospory, and adventitious embryony.
- Causes: Genetic mutations, polyploidy, epigenetic changes, hormonal imbalances, environmental stress, and evolutionary adaptations.
- Importance: Apomixis is crucial for agriculture (hybrid vigor, seed production), ecology (colonization, reproductive assurance), and evolution (genotype preservation), with potential for biotechnological advancements.
Question:-5
Enlist the categories of xerophytes. Describe the morphological and anatomical adaptations found in xerophytes.
Answer:
Categories of Xerophytes
Xerophytes are plants adapted to survive in dry environments with limited water availability, such as deserts, high altitudes, or saline soils. They are classified into distinct categories based on their strategies for coping with water scarcity. The main categories of xerophytes are:
-
Ephemeral Annuals (Drought Evaders):
- These plants complete their life cycle quickly during brief periods of moisture (e.g., after rainfall) and remain dormant as seeds during dry periods.
- Examples: Desert wildflowers like Argemone mexicana (Mexican poppy) and certain grasses.
-
Succulents (Drought Avoiders):
- These plants store water in specialized tissues (e.g., leaves, stems, or roots) to survive prolonged droughts.
- Examples: Cactus (Opuntia), Aloe vera, Agave, and Euphorbia.
-
Non-Succulent Perennials (Drought Resisters):
- These plants tolerate low water availability through physiological and structural adaptations, remaining active even in dry conditions.
- Examples: Acacia, Prosopis (mesquite), and Ziziphus (jujube).
-
Halophytes (Salt-Tolerant Xerophytes):
- A subset of xerophytes adapted to saline environments, where water is present but physiologically unavailable due to high salt concentrations.
- Examples: Atriplex (saltbush), Suaeda (sea blite), and Tamarix (tamarisk).
Morphological and Anatomical Adaptations in Xerophytes
Xerophytes exhibit a range of morphological (external structure) and anatomical (internal structure) adaptations to conserve water, reduce transpiration, and survive in arid conditions. These adaptations are described below, organized by category.
Morphological Adaptations
-
Reduced Leaf Surface Area:
- Leaves are often small, scale-like, or absent to minimize water loss through transpiration.
- Example: In Acacia, leaves are reduced to phyllodes (flattened petioles), and in cacti, leaves are modified into spines.
- Some xerophytes, like Casuarina, have needle-like leaves to reduce surface area.
-
Thick, Fleshy Structures:
- Succulents have thick, fleshy leaves, stems, or roots to store water.
- Example: Aloe vera stores water in its succulent leaves, while cacti store water in their swollen stems.
-
Sunken Stomata:
- Stomata (pores for gas exchange) are sunken into pits or grooves on the leaf surface to reduce evaporation by shielding them from dry air.
- Example: Nerium (oleander) and Agave have sunken stomata.
-
Thick Cuticle and Waxy Coating:
- A thick, waxy cuticle covers leaves and stems, reducing water loss by forming a waterproof barrier.
- Example: Euphorbia and cacti have glossy, waxy surfaces.
-
Dense Hair or Spines:
- Leaves or stems may be covered with trichomes (hairs) or spines, which reflect sunlight, reduce air flow, and trap moisture, lowering transpiration.
- Example: Cacti have spines, and Calotropis has hairy leaves.
-
Extensive Root Systems:
- Xerophytes often have long, deep taproots to access groundwater or wide, shallow roots to capture surface water after rain.
- Example: Prosopis has deep roots (up to 50 meters), while cacti have shallow, extensive root networks.
-
Stem Modifications:
- Stems may be flattened, photosynthetic, or succulent to take over the role of leaves in photosynthesis and water storage.
- Example: In Opuntia, the stem is flattened into pads (cladodes), and in Euphorbia, stems are cylindrical and succulent.
-
Shedding of Leaves or Dormancy:
- Some xerophytes shed leaves during dry periods to reduce water loss, entering dormancy until conditions improve.
- Example: Ziziphus sheds leaves seasonally.
Anatomical Adaptations
-
Thick Epidermis and Cuticle:
- The epidermis is thick with a heavy cuticle to prevent water loss.
- Example: In Nerium, the epidermal cells are multilayered with a thick cuticular layer.
-
Sunken Stomata with Guard Cell Modifications:
- Stomata are located in crypts or pits, often surrounded by trichomes, reducing exposure to dry air.
- Guard cells may be smaller and more efficient to control water loss.
- Example: Agave has stomata sunken in grooves lined with hairs.
-
Sclerenchymatous Tissue:
- Abundant sclerenchyma (thick-walled, lignified cells) in leaves and stems provides mechanical support and reduces wilting.
- Example: Acacia has sclerenchymatous fibers in its phyllodes.
-
Water-Storing Parenchyma:
- Succulents have large, thin-walled parenchyma cells in leaves or stems for water storage.
- Example: Aloe has mucilaginous parenchyma cells that store water and nutrients.
-
Reduced Vascular Tissue:
- Vascular bundles (xylem and phloem) are smaller and more compact to minimize water transport demands.
- Example: In cacti, vascular tissue is limited, with xylem vessels having narrow lumens for efficient water conduction.
-
Kranz Anatomy (in C4 Plants):
- Many xerophytes, especially grasses, exhibit C4 photosynthesis, characterized by Kranz anatomy (bundle sheath cells surrounding vascular bundles).
- This adaptation enhances water-use efficiency by concentrating CO₂, reducing the need for open stomata.
- Example: Panicum (switchgrass) and Sporobolus (dropseed).
-
Thick-Walled Mesophyll:
- The mesophyll (photosynthetic tissue) is compact with smaller intercellular spaces to reduce water loss.
- In succulents, mesophyll cells are large and specialized for water storage.
- Example: Opuntia has a multilayered, water-storing mesophyll.
-
CAM Photosynthesis:
- Many succulents use Crassulacean Acid Metabolism (CAM), where stomata open at night to take in CO₂, storing it as malic acid, and close during the day to minimize water loss.
- Example: Cactus and Agave exhibit CAM photosynthesis.
-
Root Anatomy:
- Roots have a thick cortex for water storage and a well-developed endodermis to regulate water uptake.
- Example: Prosopis roots have a thick cortex and lignified endodermal cells.
Summary of Adaptations
Adaptation Type | Morphological Examples | Anatomical Examples |
---|---|---|
Water Conservation | Sunken stomata, thick cuticle, waxy coating, dense hairs | Sunken stomata, thick epidermis, reduced vascular tissue |
Water Storage | Succulent leaves/stems, extensive roots | Water-storing parenchyma, thick cortex in roots |
Reduced Transpiration | Small/absent leaves, spines, shedding leaves | Compact mesophyll, Kranz anatomy, CAM photosynthesis |
Structural Support | Thick stems, spines | Sclerenchymatous tissue, lignified cells |
Key Points
- Categories: Xerophytes include ephemeral annuals, succulents, non-succulent perennials, and halophytes, each with unique survival strategies.
- Morphological Adaptations: Focus on reducing water loss (small leaves, sunken stomata, waxy cuticle) and storing water (succulent tissues, extensive roots).
- Anatomical Adaptations: Enhance water retention (thick epidermis, water-storing parenchyma), support (sclerenchyma), and efficiency (C4/CAM photosynthesis, Kranz anatomy).
- These adaptations enable xerophytes to thrive in arid environments, making them critical for ecological stability in deserts and potential candidates for agriculture in water-scarce regions.
Question:-6(a)
What is parthenocarpy. Describe various types of parthenocarpy found in plants.
Answer:
Definition of Parthenocarpy
Parthenocarpy is the development of fruits in plants without fertilization, resulting in seedless fruits. In parthenocarpic fruits, the ovary develops into a fruit without the formation of seeds, as the ovules either do not develop or remain unfertilized. This phenomenon can occur naturally or be induced artificially through hormonal treatments or genetic modifications. Parthenocarpy is significant in horticulture for producing seedless varieties of fruits that are more palatable and convenient for consumption.
Types of Parthenocarpy
Parthenocarpy is classified into three main types based on the mechanism and conditions under which it occurs:
-
Natural Parthenocarpy:
- Occurs spontaneously in certain plant species or cultivars without external intervention.
- Typically, these plants have genetic traits that suppress fertilization or seed development, leading to seedless fruit formation.
- Mechanism:
- The ovary develops into a fruit due to intrinsic hormonal signals (e.g., auxins, gibberellins) without pollination or fertilization.
- Ovules may degenerate or fail to develop, resulting in seedless fruits.
- Examples:
- Banana (Musa spp.): Many commercial bananas (e.g., Cavendish) are seedless due to triploidy, which prevents viable seed formation.
- Pineapple (Ananas comosus): Certain varieties produce seedless fruits naturally.
- Some grape varieties (Vitis vinifera): Seedless grapes like Thompson Seedless are naturally parthenocarpic.
- Significance: Naturally parthenocarpic plants are valued for their consistent seedless fruit production, reducing the need for pollination.
-
Induced Parthenocarpy:
- Parthenocarpy is artificially induced through external treatments, such as hormonal applications, genetic engineering, or environmental manipulations.
- Mechanism:
- Hormones like auxins, gibberellins, or cytokinins are applied to unpollinated flowers to stimulate ovary growth and fruit development.
- Genetic modifications may alter reproductive pathways to prevent fertilization while promoting fruit growth.
- Environmental factors (e.g., temperature, light) can also be manipulated to induce parthenocarpy in some species.
- Examples:
- Tomato (Solanum lycopersicum): Gibberellin sprays induce seedless fruit formation, especially in greenhouse cultivation.
- Cucumber (Cucumis sativus): Auxin treatments produce seedless cucumbers for commercial markets.
- Eggplant (Solanum melongena): Hormonal treatments can stimulate parthenocarpic fruit development.
- Significance: Induced parthenocarpy is widely used in agriculture to ensure fruit production in adverse conditions (e.g., lack of pollinators, cold weather) and to produce uniform, seedless fruits.
-
Stenospermocarpy:
- A specialized form of parthenocarpy where pollination and fertilization occur, but the embryos abort early, resulting in seedless or partially seeded fruits.
- Mechanism:
- Pollination triggers fruit development, but the developing seeds (ovules) fail to mature, either due to genetic factors or environmental stress.
- The fruit continues to grow despite the absence of viable seeds.
- Examples:
- Seedless grapes (e.g., Sultana, Crimson Seedless): Pollination occurs, but embryo abortion leads to seedless fruits.
- Certain citrus varieties (e.g., Navel oranges): Partial seed development may occur, but seeds are non-viable.
- Watermelon (Citrullus lanatus): Some seedless watermelons are produced through stenospermocarpy, often requiring pollination by a diploid pollinator.
- Significance: Stenospermocarpy bridges sexual and asexual fruit development, allowing fruit production in plants that require pollination but still yield seedless fruits.
Key Points and Comparisons
Type | Mechanism | Examples | Dependence on Pollination | Applications |
---|---|---|---|---|
Natural Parthenocarpy | Spontaneous, genetic suppression of fertilization or seed development | Banana, pineapple, some grapes | No | Consistent seedless fruit production |
Induced Parthenocarpy | Artificially stimulated by hormones, genetic engineering, or environmental factors | Tomato, cucumber, eggplant | Usually no | Commercial production in controlled settings |
Stenospermocarpy | Pollination occurs, but embryos abort, leading to seedless fruits | Seedless grapes, navel oranges | Yes | Seedless fruits with pollination requirement |
Additional Notes
- Hormonal Role: Parthenocarpy is often driven by elevated levels of plant hormones (auxins, gibberellins, cytokinins) that mimic the signals of fertilization, promoting cell division and fruit growth in the ovary.
- Genetic Basis: Parthenocarpy can result from mutations in genes controlling ovule development, fertilization, or seed formation. For example, triploidy in bananas causes sterility, leading to natural parthenocarpy.
- Agricultural Importance:
- Parthenocarpic fruits are seedless, improving consumer appeal (e.g., seedless grapes, watermelons).
- They ensure fruit production in regions with poor pollination (e.g., due to lack of pollinators or adverse weather).
- Induced parthenocarpy allows year-round fruit production in greenhouses.
- Limitations:
- Parthenocarpic fruits may be smaller or less flavorful than seeded fruits in some species.
- Induced parthenocarpy requires costly hormonal treatments or genetic engineering.
- Stenospermocarpy depends on pollination, limiting its use in pollinator-scarce environments.
Summary
- Definition: Parthenocarpy is the formation of seedless fruits without fertilization.
- Types: Natural parthenocarpy (spontaneous, genetic), induced parthenocarpy (artificially stimulated), and stenospermocarpy (pollination-dependent with embryo abortion).
- Significance: Parthenocarpy enhances fruit production, improves marketability, and supports agriculture in challenging conditions.
Question:-6(b)
Explain incompatibility and describe its types and significance.
Answer:
Explanation of Incompatibility
Incompatibility in plants refers to the physiological or genetic mechanisms that prevent successful fertilization between certain individuals, even when pollination occurs. It is a reproductive barrier that ensures outbreeding (cross-pollination) androbotics, reduces self-pollination, and promotes genetic diversity. Incompatibility systems are primarily found in flowering plants and are crucial for preventing inbreeding and maintaining genetic variation within populations. These mechanisms involve specific interactions between the pollen and the pistil that inhibit pollen tube growth or fertilization if the pollen and pistil are genetically too similar.
Incompatibility is a natural adaptation that enhances the evolutionary fitness of plants by promoting cross-pollination, which leads to greater genetic recombination and adaptability. It is controlled by specific genes, typically at the S-locus (self-incompatibility locus), which determine the compatibility between pollen and pistil.
Types of Incompatibility
Incompatibility in plants is broadly classified into two main types based on the timing and mechanism of the rejection response:
-
Gametophytic Self-Incompatibility (GSI):
- Mechanism:
- The compatibility is determined by the genotype of the pollen (gametophyte) rather than the pollen-producing parent (sporophyte).
- The pollen tube growth is inhibited in the style if the S-allele (self-incompatibility allele) of the pollen matches one of the S-alleles in the pistil.
- The rejection response occurs in the style, where the pollen tube fails to grow or grows slowly, preventing fertilization.
- Characteristics:
- Common in plants with wet stigmas and binucleate pollen (two nuclei in pollen at the time of release).
- Controlled by a single S-locus with multiple alleles (e.g., S1, S2, S3, etc.).
- The interaction involves specific proteins, such as S-RNases in the style, which degrade RNA in incompatible pollen tubes.
- Examples:
- Solanaceae (e.g., tobacco, petunia, tomato).
- Rosaceae (e.g., apple, pear, cherry).
- Papaveraceae (e.g., poppy).
- Molecular Basis:
- In Solanaceae, stylar S-RNases enter the pollen tube and degrade RNA if the pollen’s S-allele matches.
- In Papaveraceae, a calcium-mediated signaling cascade triggers programmed cell death in incompatible pollen.
- Mechanism:
-
Sporophytic Self-Incompatibility (SSI):
- Mechanism:
- The compatibility is determined by the genotype of the pollen-producing parent (sporophyte), not the pollen itself.
- The rejection response occurs on the stigma surface, where pollen germination or pollen tube penetration is inhibited if the S-alleles of the pollen parent match those of the pistil.
- Characteristics:
- Common in plants with dry stigmas and trinucleate pollen (three nuclei in pollen at release).
- Controlled by a single S-locus with multiple alleles, but the interaction is mediated by proteins on the pollen coat and stigma surface.
- The stigma expresses S-locus receptor kinases (SRKs), which interact with S-locus cysteine-rich proteins (SCRs) on the pollen coat.
- Examples:
- Brassicaceae (e.g., cabbage, mustard, radish).
- Asteraceae (e.g., sunflower, daisies).
- Molecular Basis:
- The SRK on the stigma binds to SCR on the pollen coat, triggering a signaling cascade that inhibits pollen hydration or germination.
- Mechanism:
Other Classifications of Incompatibility
In addition to GSI and SSI, incompatibility can also be categorized based on broader reproductive barriers:
-
Homomorphic Incompatibility:
- The flowers of the plant appear morphologically similar, but genetic incompatibility prevents self-fertilization.
- Both GSI and SSI fall under this category.
- Examples: Most self-incompatible angiosperms like Nicotiana (tobacco) and Brassica (mustard).
-
Heteromorphic Incompatibility:
- The flowers exhibit morphological differences (heterostyly), such as differences in style and anther lengths, which promote cross-pollination between different morphs (e.g., pin and thrum flowers).
- Incompatible pollination occurs between flowers of the same morph.
- Examples:
- Primula (primrose): Pin flowers (long style, short anthers) and thrum flowers (short style, long anthers).
- Lythrum salicaria (purple loosestrife): Tristylous with three morphs.
-
Interspecific Incompatibility:
- Incompatibility between different species, preventing hybridization.
- This can involve pollen-stigma interactions similar to SSI or GSI, or other barriers like pollen tube growth inhibition in the style.
- Examples: Incompatible crosses between Nicotiana tabacum and Nicotiana rustica.
Significance of Incompatibility
Incompatibility plays a critical role in plant reproduction, evolution, and agriculture. Its significance includes:
-
Promotion of Outbreeding:
- Incompatibility prevents self-pollination, ensuring cross-pollination between genetically distinct individuals.
- This increases genetic diversity, enhancing adaptability to changing environments and reducing inbreeding depression.
-
Evolutionary Advantage:
- By* By promoting genetic recombination, incompatibility contributes to the formation of new gene combinations, which can lead to the evolution of new traits and species.
- It helps maintain heterozygosity, improving the long-term survival of plant populations.
-
Ecological Role:
- Incompatibility supports pollinator-dependent ecosystems by requiring cross-pollination, sustaining populations of bees, butterflies, and other pollinators.
- It contributes to the genetic health of plant communities, supporting biodiversity.
-
Agricultural Importance:
- Hybrid Seed Production: Incompatibility is exploited in crops like maize, cabbage, and sorghum to produce hybrid seeds with hybrid vigor (heterosis). For example, SSI in Brassica is used to ensure cross-pollination between parental lines.
- Crop Improvement: Understanding incompatibility helps breeders develop seedless fruits (parthenocarpy) or control pollination for desired traits.
- Challenges: Incompatibility can complicate breeding programs by limiting compatible crosses, requiring techniques like bud pollination or chemical treatments to overcome barriers.
-
Conservation and Restoration:
- Incompatibility informs conservation strategies by highlighting the need for diverse pollinator populations and genetic variation in endangered plant species.
- It guides restoration projects to ensure compatible mating systems for successful reproduction.
-
Research and Biotechnology:
- Studying incompatibility provides insights into plant reproductive biology, aiding the development of genetically modified crops with controlled breeding systems.
- It helps unravel the molecular basis of plant signaling and protein interactions, advancing genetic engineering.
Summary
- Definition: Incompatibility is a genetic mechanism in plants that prevents self-fertilization, promoting outbreeding and genetic diversity.
- Types:
- Gametophytic Self-Incompatibility (GSI): Pollen tube growth inhibited in the style based on pollen genotype (e.g., Solanaceae).
- Sporophytic Self-Incompatibility (SSI): Pollen germination inhibited on the stigma based on sporophyte genotype (e.g., Brassicaceae).
- Homomorphic: Morphologically similar flowers with genetic incompatibility.
- Heteromorphic: Morphologically distinct flowers (e.g., Primula).
- Interspecific: Barriers between species.
- Significance: Enhances genetic diversity, supports hybrid seed production, sustains pollinator ecosystems, aids conservation, and informs biotechnological advancements.
Question:-7
With the help of well labeled diagram describe the ABC Model of Flower Organization.
Answer:
The ABC Model of Flower Organization
The ABC model is a genetic framework that explains the molecular basis of flower development in angiosperms (flowering plants). It describes how specific combinations of genes, known as homeotic genes, determine the identity of floral organs (sepals, petals, stamens, and carpels) in the four whorls of a flower. The model was proposed based on studies in model plants like Arabidopsis thaliana and Antirrhinum majus (snapdragon) and is widely applicable to most angiosperms.
According to the ABC model:
- Three classes of genes (A, B, and C) interact to specify the identity of floral organs.
- These genes are expressed in specific whorls of the developing flower, and their combinatorial action determines which organ develops in each whorl.
- The model also includes additional genes (e.g., E-class genes) in more refined versions to account for the complete floral structure.
Key Components of the ABC Model
-
Floral Whorls:
- A typical flower has four concentric whorls of organs, numbered from the outside to the inside:
- Whorl 1: Sepals (outermost, protective structures).
- Whorl 2: Petals (colorful, attract pollinators).
- Whorl 3: Stamens (male reproductive organs).
- Whorl 4: Carpels (female reproductive organs).
- A typical flower has four concentric whorls of organs, numbered from the outside to the inside:
-
Gene Classes:
- A-class genes: Specify sepal identity in Whorl 1 and contribute to petal identity in Whorl 2 (with B-class genes).
- Examples: APETALA1 (AP1) and APETALA2 (AP2) in Arabidopsis.
- B-class genes: Specify petal identity in Whorl 2 (with A-class genes) and stamen identity in Whorl 3 (with C-class genes).
- Examples: APETALA3 (AP3) and PISTILLATA (PI) in Arabidopsis.
- C-class genes: Specify carpel identity in Whorl 4 and stamen identity in Whorl 3 (with B-class genes).
- Example: AGAMOUS (AG) in Arabidopsis.
- E-class genes (added later): Required for the identity of all floral organs by facilitating the action of A, B, and C genes. They are expressed in all whorls.
- Example: SEPALLATA (SEP) genes in Arabidopsis.
- A-class genes: Specify sepal identity in Whorl 1 and contribute to petal identity in Whorl 2 (with B-class genes).
-
Gene Interactions:
- The ABC genes encode transcription factors (mostly MADS-box proteins) that regulate downstream genes responsible for organ development.
- The model assumes antagonistic interactions:
- A-class genes inhibit C-class genes in Whorls 1 and 2.
- C-class genes inhibit A-class genes in Whorls 3 and 4.
- The combinatorial expression of these genes determines organ identity:
- Whorl 1: A alone → Sepals.
- Whorl 2: A + B → Petals.
- Whorl 3: B + C → Stamens.
- Whorl 4: C alone → Carpels.
-
Role of E-class Genes:
- E-class genes (SEPALLATA) are expressed across all whorls and act as co-factors, enabling A, B, and C genes to form functional protein complexes.
- Without E-class genes, floral organs may develop as leaf-like structures.
Description of the ABC Model with a Well-Labeled Diagram
The ABC model can be visualized as a series of concentric whorls in a developing flower, with each whorl expressing a specific combination of A, B, and C genes.
Diagram of the ABC Model:
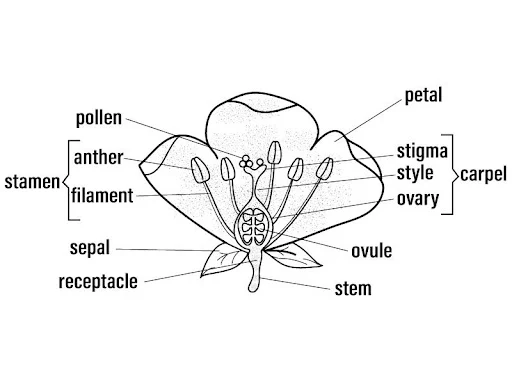
Detailed Description of a Well-Labeled Diagram:
- Structure:
- The diagram shows a cross-sectional or longitudinal view of a flower with four concentric whorls, labeled as Whorl 1 (outermost) to Whorl 4 (innermost).
- Each whorl is depicted as a ring or segment, with the corresponding floral organ (sepal, petal, stamen, carpel) illustrated.
- Labels:
- Whorl 1 (Sepals): Labeled with "A-class genes (AP1, AP2)" and an arrow indicating sepal formation. Sepals are shown as green, leaf-like structures.
- Whorl 2 (Petals): Labeled with "A-class (AP1, AP2) + B-class (AP3, PI)" and an arrow indicating petal formation. Petals are shown as colorful, broad structures.
- Whorl 3 (Stamens): Labeled with "B-class (AP3, PI) + C-class (AG)" and an arrow indicating stamen formation. Stamens are shown as filament-anther structures.
- Whorl 4 (Carpels): Labeled with "C-class (AG)" and an arrow indicating carpel formation. Carpels are shown as a central pistil with ovary, style, and stigma.
- E-class genes (SEP): Indicated as a background or overarching label across all whorls, noting their role in enabling floral organ identity.
- Gene Expression:
- Colored bars or shaded regions represent the expression of A, B, and C genes in each whorl:
- A-class: Expressed in Whorls 1 and 2 (e.g., blue shading).
- B-class: Expressed in Whorls 2 and 3 (e.g., red shading).
- C-class: Expressed in Whorls 3 and 4 (e.g., green shading).
- Overlapping regions (e.g., A+B in Whorl 2, B+C in Whorl 3) are shown with combined colors or patterns.
- Colored bars or shaded regions represent the expression of A, B, and C genes in each whorl:
- Antagonistic Interactions:
- Arrows or dashed lines indicate that A-class genes suppress C-class genes in Whorls 1 and 2, and C-class genes suppress A-class genes in Whorls 3 and 4.
- Mutant Phenotypes (optional):
- The diagram may include a side panel showing mutant flowers to illustrate the model:
- A-class mutant: Whorl 1 → Carpels, Whorl 2 → Stamens (due to C-class expression).
- B-class mutant: Whorl 2 → Sepals, Whorl 3 → Carpels (loss of petal and stamen identity).
- C-class mutant: Whorl 3 → Petals, Whorl 4 → Sepals (due to A-class expression).
- The diagram may include a side panel showing mutant flowers to illustrate the model:
Key Features of the ABC Model
-
Combinatorial Control:
- The identity of each floral organ depends on the unique combination of A, B, and C gene activities in each whorl.
- E-class genes act as "glue" to stabilize these interactions.
-
Homeotic Mutations:
- Mutations in ABC genes cause homeotic transformations, where one organ type is replaced by another:
- A-class mutants: Sepals → Carpels, Petals → Stamens.
- B-class mutants: Petals → Sepals, Stamens → Carpels.
- C-class mutants: Stamens → Petals, Carpels → Sepals.
- Mutations in ABC genes cause homeotic transformations, where one organ type is replaced by another:
-
Conserved Mechanism:
- The ABC model is conserved across angiosperms, though gene names and specific interactions may vary (e.g., DEFICIENS in snapdragon is homologous to AP3 in Arabidopsis).
-
Extensions of the Model:
- The ABCE model includes E-class genes for a complete understanding of floral development.
- D-class genes (e.g., SEEDSTICK, SHATTERPROOF) specify ovule identity within carpels but are not part of the core ABC model.
Significance of the ABC Model
- Understanding Flower Development: The model provides a genetic blueprint for how floral organs are specified, advancing developmental biology.
- Evolutionary Insights: Variations in ABC gene expression explain diverse floral morphologies across plant species (e.g., petaloid sepals in orchids).
- Agricultural Applications: Manipulating ABC genes can alter floral structures, enabling the development of novel flower shapes or seedless fruits.
- Biotechnological Potential: Genetic engineering of ABC genes can enhance ornamental plants or improve crop traits (e.g., larger petals, modified reproductive organs).
Summary
The ABC model explains how A, B, C, and E-class genes interact to specify sepal, petal, stamen, and carpel identities in the four floral whorls. A well-labeled diagram illustrates the whorls, gene expression patterns (A in Whorls 1–2, B in Whorls 2–3, C in Whorls 3–4, E in all), and resulting organs, with antagonistic interactions between A and C genes. The model is fundamental to understanding flower development and has broad applications in evolutionary biology and agriculture.
Question:-8
Describe syngamy and triple fusion in angiosperms with the help of a well labelled diagram. Enlist the major functions of endosperm in plants.
Answer:
Syngamy and Triple Fusion in Angiosperms
In angiosperms (flowering plants), fertilization involves a unique process called double fertilization, which includes two distinct events: syngamy and triple fusion. These processes occur within the embryo sac of the ovule and are critical for seed development. Below is a detailed description of both events, followed by a well-labeled diagram and the major functions of the endosperm.
Syngamy
- Definition: Syngamy is the fusion of one male gamete (sperm nucleus) with the egg cell nucleus in the embryo sac to form a diploid zygote.
- Process:
- After pollination, the pollen grain germinates on the stigma, and a pollen tube grows through the style to reach the ovule via the micropyle.
- The pollen tube delivers two haploid male gametes (sperm nuclei) into the embryo sac.
- One sperm nucleus fuses with the haploid egg cell nucleus, resulting in a diploid (2n) zygote.
- Outcome: The zygote develops into the embryo, which eventually forms the new plant.
- Significance: Syngamy ensures the formation of the embryo, the next generation of the plant, and maintains the diploid chromosome number.
Triple Fusion
- Definition: Triple fusion is the fusion of the second male gamete with the two polar nuclei in the central cell of the embryo sac to form a triploid primary endosperm nucleus (PEN).
- Process:
- The second sperm nucleus, delivered by the pollen tube, enters the central cell of the embryo sac.
- The central cell contains two haploid polar nuclei, which may fuse before or during fertilization to form a diploid polar nucleus.
- The haploid sperm nucleus fuses with the two polar nuclei (or the diploid polar nucleus), resulting in a triploid (3n) primary endosperm nucleus.
- Outcome: The primary endosperm nucleus divides mitotically to form the endosperm, a nutritive tissue that supports the developing embryo.
- Significance: Triple fusion produces the endosperm, which provides essential nutrients for embryo development and seed germination.
Double Fertilization
- Overview: Double fertilization is the combination of syngamy and triple fusion, a hallmark of angiosperms.
- Steps:
- The pollen tube enters the embryo sac through the micropyle, often guided by the synergids.
- The pollen tube releases two sperm nuclei into the embryo sac.
- One sperm nucleus undergoes syngamy with the egg cell (forming the zygote).
- The other sperm nucleus undergoes triple fusion with the polar nuclei (forming the primary endosperm nucleus).
- Result: The ovule develops into a seed, with the zygote forming the embryo and the primary endosperm nucleus forming the endosperm.
Well-Labeled Diagram of Syngamy and Triple Fusion
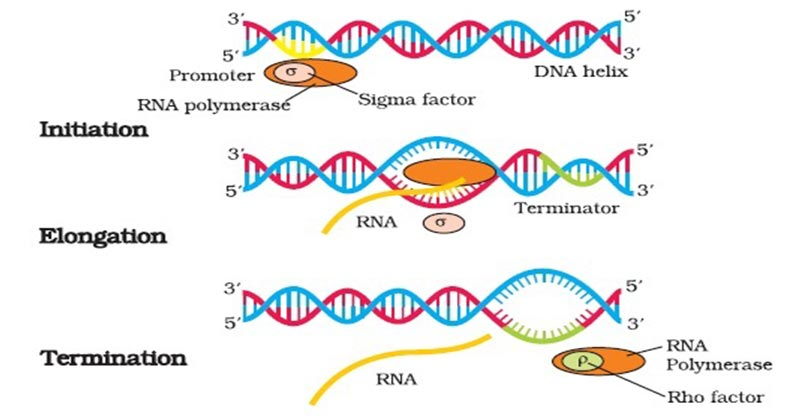
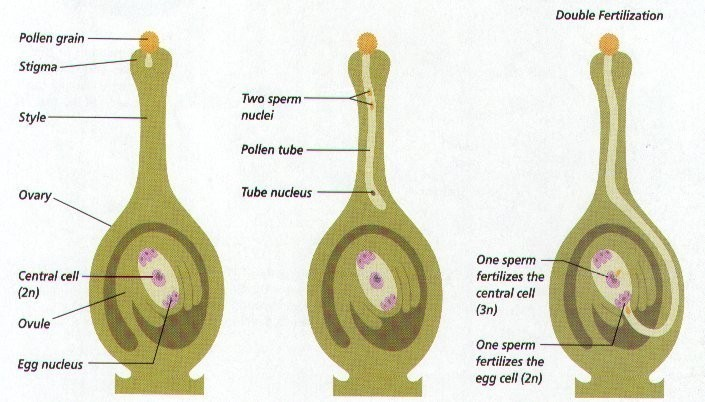
Labels:
- Pollen Tube: Enters via the micropyle, carrying two sperm nuclei.
- Egg Cell: Haploid cell that fuses with one sperm nucleus (labeled "Syngamy").
- Zygote (2n): Result of syngamy, develops into the embryo.
- Central Cell: Contains two polar nuclei, which fuse with the second sperm nucleus (labeled "Triple Fusion").
- Primary Endosperm Nucleus (PEN, 3n): Result of triple fusion, develops into the endosperm.
- Synergids: Assist in guiding the pollen tube, often degenerate after fertilization.
- Antipodal Cells: Three cells at the chalazal end, with unclear function.
- Micropylar End: Entry point for the pollen tube.
- Chalazal End: Opposite end, near the ovule’s vascular supply.
Major Functions of Endosperm in Plants
The endosperm is a triploid tissue formed by triple fusion and serves as a critical component of the seed. Its major functions include:
-
Nutrient Storage and Supply:
- The endosperm stores nutrients such as starch, proteins, lipids, and sugars, which are essential for the developing embryo.
- It provides energy and building blocks for embryo growth during seed development and germination.
- Example: In cereals like maize and wheat, the endosperm is the primary storage tissue, forming the bulk of the grain.
-
Support for Embryo Development:
- The endosperm supplies nutrients to the embryo during its early stages, ensuring proper cell division and differentiation.
- It acts as a temporary food source until the embryo develops its own photosynthetic capacity post-germination.
- Example: In beans, the endosperm is consumed by the embryo during seed maturation, leaving large cotyledons.
-
Regulation of Seed Germination:
- The endosperm produces enzymes (e.g., amylases, proteases) that break down stored nutrients into usable forms during germination.
- It may also release hormones or signaling molecules that trigger germination processes.
- Example: In barley, the endosperm releases gibberellins to initiate germination.
-
Protection of the Embryo:
- The endosperm acts as a physical and biochemical barrier, protecting the embryo from mechanical damage, pathogens, and environmental stress.
- In some seeds, the endosperm hardens (e.g., in cereals) to form a protective layer.
-
Contribution to Seed Size and Viability:
- The size and nutrient content of the endosperm influence seed size, which affects seedling vigor and survival.
- A well-developed endosperm enhances seed viability and competitive ability in natural and agricultural settings.
- Example: Large endosperms in rice and corn seeds support robust seedling establishment.
-
Economic Importance:
- The endosperm is a major source of food for humans and animals, as it constitutes the edible portion of many seeds.
- Example: The endosperm in wheat (flour), rice, and corn is used for food products, while in coconut, the endosperm forms both the liquid (coconut water) and solid (coconut meat).
Question:-9
Differentiate between: i) Porous and non-porous wood ii) Dicot stem and monocot stem iii) Open and closed style iv) Heartwood and sapwood v) Glandular and non-glandular trichomes
Answer:
i) Porous vs. Non-Porous Wood:
- Porous Wood: Contains vessel elements, allowing water transport, typical in hardwoods (dicots). It has distinct growth rings and pores (e.g., oak, teak). Often denser and used in furniture.
- Non-Porous Wood: Lacks vessels, relying on tracheids for transport, typical in softwoods (gymnosperms). It appears uniform, with no visible pores (e.g., pine, cedar). Used in construction.
ii) Dicot Stem vs. Monocot Stem:
- Dicot Stem: Has vascular bundles arranged in a ring, with distinct cortex, pith, and cambium for secondary growth. Example: sunflower stem, showing clear tissue organization.
- Monocot Stem: Vascular bundles are scattered, with no cambium, limiting secondary growth. Example: maize stem, with uniform parenchyma and no distinct pith.
iii) Open vs. Closed Style (in floral morphology):
- Open Style: The style (part of the pistil) has a canal or groove for pollen tube growth, common in some angiosperms (e.g., grasses). Facilitates easier pollen tube passage.
- Closed Style: The style is solid, with a core of transmitting tissue for pollen tubes, typical in many dicots (e.g., roses). Provides structured guidance for pollen.
iv) Heartwood vs. Sapwood:
- Heartwood: The central, older, non-living wood, darker due to resins and tannins, providing structural support (e.g., dark core in walnut). Resistant to decay.
- Sapwood: The outer, living wood, lighter in color, conducts water and nutrients (e.g., light outer layers in pine). More susceptible to pests.
v) Glandular vs. Non-Glandular Trichomes:
- Glandular Trichomes: Hair-like structures secreting substances like oils or resins, aiding defense or attraction (e.g., mint leaves’ oil-secreting trichomes).
- Non-Glandular Trichomes: Non-secretory, serving physical protection or reducing water loss (e.g., tomato leaf trichomes, deterring herbivores).
Each pair highlights distinct structural or functional adaptations in plants, critical for their survival and ecological roles.
Question:-10
Write short notes on: i) Aerial roots ii) Rhizomes iii) Cambial variant in stems iv) Dendrochronology
Answer:
i) Aerial Roots: Aerial roots are roots that grow above the ground, often in plants adapted to specific environments. They serve functions like anchorage, nutrient absorption, or gas exchange. For example, epiphytes like orchids develop aerial roots to absorb moisture from the air, while mangroves use stilt-like aerial roots for stability in muddy soils and oxygen uptake in waterlogged conditions. These roots often have specialized tissues, like velamen radicum in orchids, for water retention.
ii) Rhizomes: Rhizomes are underground, horizontal stems that store nutrients and enable vegetative reproduction. They produce shoots and roots, allowing plants to spread. Examples include ginger and turmeric, where rhizomes are harvested for culinary use. Rhizomes help plants survive adverse conditions by storing energy and regenerating after dormancy, contributing to their resilience in ecosystems like grasslands.
iii) Cambial Variant in Stems: Cambial variants refer to deviations from typical secondary growth in stems, seen in some plants like lianas or desert species. Examples include anomalous secondary growth, where cambium produces irregular vascular tissues, as in Bougainvillea (forming multiple vascular cylinders) or succulents (producing corky layers). These adaptations enhance flexibility, water storage, or structural support in extreme environments.
iv) Dendrochronology: Dendrochronology is the science of dating tree rings to study past environmental conditions and chronological events. Each ring represents a year’s growth, with width and density reflecting climate factors like rainfall or temperature. For example, narrow rings in pine trees may indicate drought years. It’s used in archaeology (e.g., dating wooden structures), climatology, and ecology to reconstruct historical environmental patterns with high precision.