BZYET-141 Solved Assignment
-
a) List various innate immune system barriers and protection mechanisms.
b) Give a brief account of the secondary lymphoid organs of the Immune System. -
a) What is immune deficiency? Explain how our immune system prevents autoimmunity?
b) What are the different types of hypersensitivity, according to Gell and Coombs? Briefly explain. -
Define the following terms:
i) Mucosa Associated Lymphoid Tissue (MALT)
ii) Haematopoiesis
iii) Hinge region of the antibody
iv) Human Leucocyte Antigen (HLA) -
Write short notes on the following:
i) Monoclonal antibodies
ii) Opsonization
iii) DNA Vaccines
iv) Agglutination Reactions -
Differentiate between the following pairs of terms:
i) Helper "T T " cells and cytotoxic "T T " cells
ii) Active and Passive immunity
iii) MHC class-I and II molecules
iv) Self and non-self antigens -
What is the vaccine? Explain the mode of action of vaccine. Write about the advantages and disadvantages of vaccination.
-
a) Write the various steps in the establishment of bacterial infection.
b) Explain the neutralization mechanism of bacteria by antibodies. -
a) Define autoimmunity. Describe any two autoimmune disorders.
b) Describe the steps involved in the immunoelectrophoresis technique.
9. a) Define antibodies and draw the structure of immunoglobulin.
b) Explain the significance of the different classes of antibodies.
10. How is the classical complement pathway activated? Discuss with a suitable diagram.
9. a) Define antibodies and draw the structure of immunoglobulin.
b) Explain the significance of the different classes of antibodies.
10. How is the classical complement pathway activated? Discuss with a suitable diagram.
Answer:
Question:-1
a) List various innate immune system barriers and protection mechanisms.
Answer:
The innate immune system is the body’s first line of defense against pathogens, providing immediate but nonspecific protection. It involves various physical, chemical, and cellular mechanisms designed to prevent or limit infection. Below is a comprehensive list of the key innate immune system barriers and protection mechanisms:
1. Physical Barriers
Physical barriers act as the first line of defense by preventing the entry of pathogens into the body.
- Skin: The outermost layer of the skin, the epidermis, acts as a tough, impermeable barrier to microorganisms. Its keratinized surface is difficult for bacteria, viruses, and fungi to penetrate.
- Mucous Membranes: The lining of body cavities that open to the outside (e.g., respiratory, gastrointestinal, and urogenital tracts) produces mucus, which traps pathogens. These membranes also contain cilia that help expel microorganisms from the body (e.g., in the respiratory tract).
- Coughing and Sneezing: Reflex actions like coughing and sneezing help expel pathogens from the respiratory tract.
2. Chemical Barriers
Chemical barriers help prevent pathogen growth and survival on surfaces, adding another layer of defense.
- Sebum: Produced by sebaceous glands in the skin, sebum contains fatty acids that inhibit microbial growth and provide a slightly acidic environment.
- Acidic pH: The skin, stomach, and vagina have an acidic pH that is hostile to many microorganisms. For instance, stomach acid (gastric acid) kills most ingested pathogens.
- Lysozyme: An enzyme found in tears, saliva, and mucus, lysozyme breaks down bacterial cell walls, leading to the destruction of certain bacteria.
- Defensins: These small peptides, present in various bodily fluids and tissues, disrupt the membranes of bacteria, fungi, and viruses.
- Acidic Urine: The low pH of urine helps prevent the growth of pathogens in the urinary tract.
3. Cellular Barriers
Various immune cells play a crucial role in identifying and neutralizing pathogens as part of the innate immune response.
-
Phagocytes: These are immune cells that engulf and digest pathogens, dead cells, and debris.
- Macrophages: Found throughout the body, macrophages recognize pathogens via pattern recognition receptors (PRRs), such as Toll-like receptors (TLRs), and ingest them.
- Neutrophils: The most abundant white blood cells, neutrophils quickly respond to infections, migrating to the site of infection and engulfing microbes.
- Dendritic Cells: These cells are important for both the innate immune response and the initiation of the adaptive immune response. They act as antigen-presenting cells that can trigger adaptive immunity after phagocytosis.
-
Natural Killer (NK) Cells: NK cells are a subset of lymphocytes that recognize and kill infected or tumor cells by inducing apoptosis (programmed cell death). They are effective against certain viral and cancerous cells.
4. Inflammatory Response
The inflammatory response is activated when tissue is injured or infected, and it is a key mechanism of the innate immune system.
- Vasodilation: Inflammation causes the blood vessels near the site of infection to dilate, increasing blood flow, which allows more immune cells (e.g., neutrophils and macrophages) to reach the site.
- Increased Vascular Permeability: The permeability of blood vessels increases, allowing immune cells and proteins like antibodies and clotting factors to pass into the affected tissue.
- Cytokines and Chemokines: These signaling molecules are released during inflammation and attract immune cells to the site of infection. Interleukins, tumor necrosis factor (TNF), and interferons are examples of cytokines involved in the inflammatory response.
5. Complement System
The complement system consists of a series of proteins found in blood plasma that, when activated, can directly kill pathogens or mark them for destruction by other immune cells.
- Classical Pathway: This is activated by the binding of antibodies to pathogens, leading to the formation of a complex that promotes pathogen destruction.
- Alternative Pathway: This pathway is triggered by pathogen surfaces and leads to the formation of a membrane attack complex (MAC) that creates holes in the pathogen’s membrane, causing lysis.
- Lectin Pathway: This pathway is activated by lectins (carbohydrate-binding proteins) that bind to sugar molecules on pathogens, leading to complement activation.
- Opsonization: Complement proteins bind to the surface of pathogens, tagging them for phagocytosis by immune cells.
6. Acute-Phase Proteins
Acute-phase proteins are produced by the liver in response to infection or injury and help enhance the immune response.
- C-reactive protein (CRP): CRP levels rise during infections and act as an opsonin to help phagocytes recognize and destroy pathogens.
- Fibrinogen: This protein plays a key role in blood clotting and helps form fibrin clots to limit the spread of pathogens.
- Mannose-binding lectin (MBL): This protein binds to sugars on the surface of pathogens, promoting complement activation and pathogen clearance.
7. Interferons
Interferons are signaling proteins released by virus-infected cells to alert neighboring cells to the presence of a viral infection.
- Type I Interferons (e.g., interferon-alpha, interferon-beta): These are released by infected cells and bind to receptors on neighboring cells, inducing the expression of antiviral proteins that inhibit viral replication.
- Type II Interferon (interferon-gamma): Produced by immune cells like NK cells and T lymphocytes, interferon-gamma enhances the ability of phagocytes to destroy pathogens.
8. Fever
Fever is a systemic response that typically occurs during infections. It is regulated by the hypothalamus in response to pyrogens (fever-inducing substances such as bacterial toxins and cytokines).
- Temperature Increase: A higher body temperature creates an environment less favorable for the growth and replication of many pathogens.
- Immune Activation: Fever also enhances the activity of immune cells, such as phagocytes, and increases the production of certain immune molecules like interferons.
9. Molecular Pattern Recognition
Innate immune cells recognize conserved molecular patterns found on pathogens but not on host cells. These patterns, known as pathogen-associated molecular patterns (PAMPs), include molecules like lipopolysaccharides (LPS) and peptidoglycan found in bacteria.
- Pattern Recognition Receptors (PRRs): PRRs, such as Toll-like receptors (TLRs) and NOD-like receptors (NLRs), are expressed on the surface or inside of immune cells. These receptors detect PAMPs and trigger the activation of immune responses, including the release of cytokines and the recruitment of immune cells.
10. The Role of the Gut Microbiota
The gut microbiota (the collection of microorganisms living in the intestines) plays an important role in supporting the innate immune system by outcompeting harmful pathogens.
- Competitive Exclusion: The normal microbiota competes with pathogenic microbes for nutrients and space, preventing pathogen colonization.
- Production of Antimicrobial Compounds: Certain beneficial microbes produce substances like bacteriocins that directly inhibit the growth of harmful bacteria.
Conclusion
The innate immune system utilizes a multifaceted approach to defend the body against infections, relying on physical, chemical, and cellular barriers as well as complex molecular mechanisms like inflammation, the complement system, and interferons. These systems work together to rapidly detect and neutralize pathogens before the adaptive immune system is activated. Each component of the innate immune system is critical for providing robust, immediate defense, helping to prevent infection and maintaining health.
The innate immune system utilizes a multifaceted approach to defend the body against infections, relying on physical, chemical, and cellular barriers as well as complex molecular mechanisms like inflammation, the complement system, and interferons. These systems work together to rapidly detect and neutralize pathogens before the adaptive immune system is activated. Each component of the innate immune system is critical for providing robust, immediate defense, helping to prevent infection and maintaining health.
b) Give a brief account of the secondary lymphoid organs of the Immune System.
Answer:
Secondary lymphoid organs are essential components of the immune system where immune cells are activated and where immune responses are initiated. Unlike primary lymphoid organs (like the bone marrow and thymus), which are responsible for the production and maturation of immune cells, secondary lymphoid organs are involved in the maturation, activation, and coordination of immune responses. These organs facilitate the interaction between immune cells and foreign antigens. The primary secondary lymphoid organs include:
1. Lymph Nodes
Lymph nodes are distributed throughout the body, especially in the neck, armpits, groin, and along major blood vessels. They are small, bean-shaped structures that act as filtering stations for lymph fluid.
- Structure: Lymph nodes are composed of an outer cortex, containing B cells, and an inner medulla, where T cells and other immune cells are found.
- Function: Lymph nodes filter lymph fluid from surrounding tissues, trapping pathogens, debris, and foreign particles. They are sites where B cells can encounter antigens and differentiate into plasma cells, while T cells can be activated to respond to pathogens.
2. Spleen
The spleen is the largest secondary lymphoid organ, located in the upper left abdomen. It serves as a critical site for filtering blood and mounting immune responses.
- Structure: The spleen consists of two main regions: the white pulp, which contains immune cells such as B cells and T cells, and the red pulp, which is involved in filtering blood and recycling iron from red blood cells.
- Function: The spleen filters blood by removing old or damaged red blood cells and pathogens. It is also a major site for the activation of immune responses, especially against blood-borne pathogens.
3. Mucosa-Associated Lymphoid Tissue (MALT)
MALT is a collection of lymphoid tissues found in mucosal surfaces that line the respiratory, gastrointestinal, and urogenital tracts. It includes several specific structures:
-
Tonsils: Lymphoid tissues located in the pharyngeal region that help protect against pathogens entering through the mouth or nose.
-
Peyer’s Patches: Located in the small intestine, these structures contain clusters of lymphoid follicles that help defend against gastrointestinal pathogens.
-
Appendix: The appendix is a lymphoid organ located at the junction of the small and large intestines, believed to play a role in immune surveillance in the gastrointestinal system.
-
Function: MALT serves as the first line of defense against pathogens that enter through mucosal surfaces. It helps initiate immune responses to pathogens in the respiratory, digestive, and urogenital systems, where most infections begin.
4. Lymphatic Vessels
While not an organ per se, lymphatic vessels are a key part of the secondary lymphoid system. They transport lymph (a fluid containing immune cells, waste products, and pathogens) between the tissues and lymph nodes.
- Function: Lymphatic vessels provide the means for immune cells to travel between secondary lymphoid organs and to areas of infection or injury, allowing rapid immune responses.
Conclusion
Secondary lymphoid organs are crucial for maintaining immune surveillance and initiating effective immune responses. They provide environments where immune cells can encounter pathogens, become activated, and coordinate the defense against infections. By filtering lymph and blood, as well as sampling antigens from mucosal surfaces, these organs play a pivotal role in the body’s ability to fight infections.
Question:-2
a) What is immune deficiency? Explain how our immune system prevents autoimmunity?
Answer:
1. Introduction to Immune Deficiency
Immune deficiency refers to a condition in which the immune system’s ability to respond to pathogens is impaired, resulting in increased susceptibility to infections, diseases, or even cancer. The immune system, a complex network of cells, proteins, and organs, is essential for defending the body against harmful microorganisms like bacteria, viruses, and fungi. When this defense system fails to function properly, either because of genetic factors or external factors (such as infections or medications), the body becomes vulnerable to various illnesses.
Immune deficiency can be classified into two main categories: primary (congenital) immune deficiency and secondary (acquired) immune deficiency. Primary immune deficiencies are typically inherited and result from genetic mutations that impair immune function. Secondary immune deficiencies, on the other hand, are acquired and can be caused by factors such as infections (e.g., HIV), malnutrition, or medical treatments like chemotherapy or immunosuppressive drugs.
2. Causes of Immune Deficiency
Immune deficiency can arise from various causes that affect different components of the immune system.
-
Genetic Mutations: Primary immune deficiencies often stem from inherited genetic mutations that affect the development or function of immune cells. Examples include severe combined immunodeficiency (SCID), in which both T cells and B cells are affected, or X-linked agammaglobulinemia (XLA), a condition that leads to a lack of mature B cells.
-
Infections: Certain infections, especially HIV, can lead to acquired immune deficiency. HIV attacks CD4+ T cells, a type of white blood cell crucial for immune function, progressively weakening the immune system and eventually leading to AIDS (Acquired Immunodeficiency Syndrome).
-
Medications: Immunosuppressive drugs, often used in organ transplants or to treat autoimmune diseases, can suppress the immune system’s ability to fight infections. Chemotherapy, used to treat cancer, also weakens the immune system by killing rapidly dividing cells, including immune cells.
-
Malnutrition: Adequate nutrition is vital for maintaining a strong immune system. Malnutrition, especially deficiencies in vitamins and minerals like vitamin A, C, D, and zinc, can compromise immune function and make the body more susceptible to infections.
-
Environmental Factors: Chronic exposure to certain toxins, chemicals, or radiation can also weaken the immune system.
3. Types of Immune Deficiency
There are several types of immune deficiencies, and they are broadly categorized into primary and secondary types.
-
Primary Immunodeficiencies: These are rare and typically caused by genetic mutations affecting immune cells or proteins.
- Humoral Immunodeficiencies: These impair B cells and their ability to produce antibodies, making the body more susceptible to bacterial infections.
- Cellular Immunodeficiencies: These affect T cells, which play a key role in responding to viral infections and in regulating immune responses.
- Combined Immunodeficiencies: These affect both B and T cells, leading to severe immunodeficiency, as seen in conditions like SCID.
-
Secondary Immunodeficiencies: These are more common and result from environmental or external factors.
- HIV/AIDS: A viral infection that causes immune system dysfunction by targeting and destroying CD4+ T cells.
- Medications and Cancer Treatment: Drugs or treatments that suppress the immune system, leaving individuals vulnerable to infections.
- Chronic Diseases: Conditions like diabetes or cancer can lead to weakened immune responses, increasing susceptibility to infections.
4. How the Immune System Prevents Autoimmunity
Autoimmunity occurs when the immune system mistakenly attacks the body’s own cells and tissues, perceiving them as foreign invaders. This can lead to a variety of autoimmune diseases, such as rheumatoid arthritis, lupus, and multiple sclerosis. The immune system has several mechanisms in place to prevent autoimmunity and maintain tolerance to the body’s own tissues.
Central Tolerance
Central tolerance is established during the early stages of immune cell development, primarily in the thymus for T cells and the bone marrow for B cells. During this process, immune cells that recognize self-antigens too strongly are eliminated, ensuring that only those that recognize foreign antigens are allowed to mature and become functional.
-
T Cell Negative Selection: In the thymus, developing T cells undergo a process called negative selection, where any T cell that strongly binds to self-antigens presented by thymic cells is induced to die (apoptosis). This prevents the release of self-reactive T cells into circulation.
-
B Cell Negative Selection: In the bone marrow, developing B cells that bind to self-antigens are either eliminated or rendered inactive. This ensures that mature B cells will produce antibodies only against non-self antigens.
Peripheral Tolerance
Despite central tolerance, some self-reactive immune cells may still escape into the peripheral circulation. To prevent these cells from causing autoimmune reactions, the immune system has several mechanisms of peripheral tolerance.
-
Regulatory T Cells (Tregs): Tregs are specialized T cells that play a crucial role in suppressing immune responses. They can inhibit the activation of self-reactive T cells, preventing them from attacking the body’s own tissues. Tregs maintain immune homeostasis by recognizing self-antigens and regulating immune responses to prevent autoimmunity.
-
Clonal Anergy: This occurs when self-reactive T or B cells encounter self-antigens but fail to receive the necessary co-stimulatory signals required for full activation. As a result, these cells become anergic (inactive) and are unable to mount an immune response against self-tissues.
-
Activation-Induced Cell Death (AICD): Self-reactive T cells that become activated in the presence of self-antigens undergo programmed cell death (apoptosis). This mechanism ensures that once immune cells respond to a self-antigen, they do not persist and cause damage to healthy tissues.
-
Checkpoint Inhibitors: Immune checkpoints are molecules that regulate immune responses to prevent overactivity. For example, the PD-1/PD-L1 pathway helps prevent T cells from attacking self-tissues by inhibiting their activation once they recognize self-antigens.
Immune Privilege Sites
Certain areas of the body, such as the eyes, brain, and testes, are considered "immune-privileged" sites. These areas have specialized mechanisms that reduce the likelihood of an autoimmune response occurring within them.
-
Blood-Brain Barrier: The blood-brain barrier restricts the entry of immune cells into the brain, limiting the chances of autoimmunity in the central nervous system.
-
Immunosuppressive Cytokines: Immune-privileged sites often produce immunosuppressive cytokines that dampen immune responses and prevent inflammation, reducing the likelihood of tissue damage from autoimmune attacks.
5. Autoimmune Disease and Breakdown of Tolerance
Despite these protective mechanisms, autoimmune diseases can occur when tolerance breaks down. Factors that contribute to the development of autoimmune diseases include:
-
Genetic Predisposition: Certain genetic factors, such as specific alleles of the human leukocyte antigen (HLA) genes, increase the risk of autoimmune diseases by altering immune cell recognition of self-antigens.
-
Environmental Triggers: Infections, drugs, or toxins can trigger autoimmune responses by causing changes in self-antigens or by stimulating immune cells in ways that promote autoimmunity. For example, viral infections may mimic self-antigens, leading to molecular mimicry, where the immune system mistakenly targets both the pathogen and the body’s own tissues.
-
Failure of Regulatory Mechanisms: If mechanisms like regulatory T cell function or checkpoint inhibition are impaired, self-reactive immune cells may be able to attack the body’s tissues, leading to autoimmune diseases.
6. Conclusion
Immune deficiency refers to the impaired ability of the immune system to defend the body against pathogens, resulting in increased susceptibility to infections and diseases. It can be primary (genetic) or secondary (acquired), with various causes such as infections, medications, or malnutrition. The immune system has several sophisticated mechanisms in place to prevent autoimmunity, including central and peripheral tolerance, regulatory T cells, and immune checkpoint pathways. However, when these mechanisms fail due to genetic factors, environmental triggers, or other disruptions, autoimmune diseases can arise. Understanding the mechanisms of immune deficiency and autoimmunity is crucial for developing therapies and interventions to restore immune function and prevent the harmful effects of autoimmunity.
b) What are the different types of hypersensitivity, according to Gell and Coombs? Briefly explain.
Answer:
1. Introduction to Hypersensitivity
Hypersensitivity refers to an exaggerated or inappropriate immune response to a substance (allergen) that does not typically cause harm in most people. These responses lead to tissue damage and can manifest in various forms, ranging from mild allergic reactions to severe, life-threatening conditions. The classification of hypersensitivity reactions was introduced by Gell and Coombs in 1963, who categorized them into four types based on the immunological mechanisms involved. Understanding these types helps in diagnosing, treating, and managing allergic and immune-mediated disorders.
2. Type I Hypersensitivity: Immediate or Anaphylactic Hypersensitivity
Type I hypersensitivity, also known as immediate hypersensitivity, is an allergic reaction that occurs quickly after exposure to an allergen. This is the most common type of hypersensitivity and involves the activation of IgE antibodies and mast cells.
-
Mechanism: When an individual is sensitized to an allergen (e.g., pollen, pet dander, or certain foods), the immune system produces IgE antibodies specific to that allergen. Upon subsequent exposure, the allergen binds to the IgE on the surface of mast cells and basophils, triggering the release of histamine and other inflammatory mediators. These mediators cause the symptoms associated with allergic reactions, such as swelling, redness, itching, and bronchoconstriction.
-
Clinical Manifestations: Type I hypersensitivity can manifest in a variety of ways, including:
- Allergic rhinitis (hay fever): Sneezing, nasal congestion, and itching.
- Asthma: Wheezing, shortness of breath, and coughing due to bronchoconstriction.
- Urticaria (hives): Raised, red welts on the skin.
- Anaphylaxis: A severe, systemic reaction that can lead to shock, respiratory failure, and even death if untreated. It requires immediate medical intervention, typically with epinephrine.
-
Treatment: Antihistamines, corticosteroids, and allergen immunotherapy (allergy shots) can help manage Type I hypersensitivity. In severe cases, epinephrine is used to reverse anaphylactic reactions.
3. Type II Hypersensitivity: Antibody-Mediated Hypersensitivity
Type II hypersensitivity, also called cytotoxic hypersensitivity, occurs when antibodies (mainly IgG or IgM) bind to antigens present on the surface of host cells, leading to cell damage and destruction. This type of reaction typically involves complement activation or antibody-dependent cell-mediated cytotoxicity (ADCC).
-
Mechanism: In Type II hypersensitivity, antibodies recognize specific antigens on the surface of host cells (such as red blood cells, platelets, or tissue cells). The immune system then mounts a response that can destroy these cells through the activation of the complement system or phagocytosis by macrophages. This can result in cell lysis or dysfunction.
-
Clinical Manifestations: Common conditions associated with Type II hypersensitivity include:
- Autoimmune hemolytic anemia: Destruction of red blood cells leading to anemia.
- Blood transfusion reactions: If a person receives an incompatible blood type, antibodies attack the transfused red blood cells, leading to hemolysis.
- Goodpasture syndrome: An autoimmune disorder where antibodies attack the basement membrane of the lungs and kidneys, leading to renal and pulmonary damage.
- Graves’ disease and Myasthenia gravis: Both are examples of autoimmune disorders where antibodies activate or inhibit normal cellular functions, leading to overactive or underactive organs.
-
Treatment: The management of Type II hypersensitivity typically involves removing or neutralizing the offending antibodies (e.g., using plasmapheresis or immunosuppressive therapies) and addressing the symptoms of organ damage.
4. Type III Hypersensitivity: Immune Complex-Mediated Hypersensitivity
Type III hypersensitivity, also known as immune complex-mediated hypersensitivity, occurs when antigen-antibody complexes (immune complexes) deposit in tissues and provoke inflammation and tissue damage. The immune complexes consist of antigens (from pathogens or self-tissues) and antibodies, primarily IgG or IgM.
-
Mechanism: When antigen-antibody complexes form in the bloodstream, they can deposit in various tissues, such as the kidneys, joints, and blood vessels. These deposits trigger the activation of the complement system and recruit neutrophils to the site of deposition. The subsequent release of inflammatory mediators (e.g., proteases and reactive oxygen species) leads to tissue injury, inflammation, and the development of disease.
-
Clinical Manifestations: Type III hypersensitivity is associated with diseases that involve systemic inflammation and tissue damage. Common examples include:
- Systemic lupus erythematosus (SLE): A systemic autoimmune disease in which immune complexes deposit in the kidneys, joints, and skin, leading to nephritis, arthritis, and rashes.
- Rheumatoid arthritis: Chronic inflammation of the joints due to immune complex deposition.
- Serum sickness: A reaction that occurs after receiving certain medications (e.g., antiserum), where immune complexes deposit in tissues and cause fever, rashes, and joint pain.
- Polyarteritis nodosa: A vasculitis in which immune complexes deposit in blood vessel walls, causing inflammation and damage.
-
Treatment: Type III hypersensitivity reactions are treated with anti-inflammatory drugs, immunosuppressive medications, and plasma exchange in severe cases to remove circulating immune complexes.
5. Type IV Hypersensitivity: Cell-Mediated or Delayed-Type Hypersensitivity
Type IV hypersensitivity, also known as delayed-type hypersensitivity (DTH), is mediated by T cells rather than antibodies. It involves the activation of CD4+ T helper cells and CD8+ cytotoxic T cells, leading to inflammation and tissue damage. This response typically occurs 24-72 hours after exposure to the antigen, hence the term "delayed."
-
Mechanism: In Type IV hypersensitivity, T cells recognize and respond to an antigen, releasing cytokines that recruit and activate macrophages and other immune cells to the site of exposure. This leads to tissue damage due to the release of inflammatory cytokines, enzymes, and free radicals by macrophages. Unlike other hypersensitivity types, there are no antibodies involved.
-
Clinical Manifestations: Type IV hypersensitivity reactions are associated with chronic inflammatory conditions. Examples include:
- Contact dermatitis: A skin reaction caused by exposure to allergens like poison ivy, nickel, or certain chemicals. It involves CD4+ T cell activation and macrophage infiltration.
- Tuberculin skin test (Mantoux test): A diagnostic test for tuberculosis that induces a localized Type IV hypersensitivity response in individuals previously exposed to Mycobacterium tuberculosis.
- Chronic transplant rejection: A delayed immune response to a transplanted organ, where T cells recognize the transplanted tissue as foreign and mount an immune response against it.
-
Treatment: Type IV hypersensitivity is typically managed with topical corticosteroids, systemic immunosuppressive agents, and antihistamines in certain cases. The primary focus is to reduce inflammation and control symptoms.
6. Conclusion
Hypersensitivity reactions, as categorized by Gell and Coombs, are classified into four types based on the immune mechanisms involved. Type I hypersensitivity is mediated by IgE and mast cells and includes common allergic reactions like asthma and anaphylaxis. Type II hypersensitivity involves antibody-mediated destruction of cells, as seen in autoimmune hemolytic anemia and transfusion reactions. Type III hypersensitivity is characterized by immune complex deposition and inflammation, leading to diseases like lupus and rheumatoid arthritis. Lastly, Type IV hypersensitivity is a cell-mediated response, involving T cells and macrophages, and causes delayed reactions such as contact dermatitis and chronic transplant rejection. Understanding these types is crucial for diagnosing, treating, and managing allergic and autoimmune diseases, helping improve patient care and outcomes.
Question:-3
a) Define Mucosa Associated Lymphoid Tissue (MALT).
Answer:
i) Mucosa-Associated Lymphoid Tissue (MALT)
Mucosa-Associated Lymphoid Tissue (MALT) refers to the collection of lymphoid tissues found in the mucosal linings of the body, such as in the gastrointestinal (GI), respiratory, and urogenital tracts. MALT is a key component of the immune system and plays a crucial role in protecting the body from pathogens that enter through mucosal surfaces. It includes lymphoid structures such as tonsils, Peyer’s patches (in the small intestine), appendix, and other diffuse lymphoid tissues. These structures house immune cells like B cells, T cells, macrophages, and dendritic cells that help identify and respond to foreign antigens, providing localized immune defense and contributing to the development of immune tolerance. MALT is essential for the first line of defense against infections at mucosal surfaces.
b) Define Haematopoiesis.
Answer:
ii) Haematopoiesis
Haematopoiesis is the process of blood cell formation and development, occurring primarily in the bone marrow in adults and in the liver and spleen during fetal development. This process produces all the different types of blood cells, including red blood cells (erythrocytes), white blood cells (leukocytes), and platelets (thrombocytes). Haematopoiesis begins with hematopoietic stem cells (HSCs), which are multipotent stem cells capable of differentiating into various blood cell lineages. The process is regulated by various cytokines and growth factors, such as erythropoietin for red blood cells and colony-stimulating factors for white blood cells. Haematopoiesis is a dynamic process that ensures the continual production and replenishment of blood cells throughout an individual’s lifetime.
c) Define the Hinge region of the antibody.
Answer:
iii) Hinge Region of the Antibody
The hinge region of an antibody is a flexible segment of the protein structure located between the Fab (antigen-binding) and Fc (constant) regions of the antibody. It plays a critical role in allowing the antibody to move and adapt its shape, facilitating binding to antigens and immune cell receptors. The hinge region is composed of a series of amino acids and provides structural flexibility that allows the antibody’s Fab arms (the two antigen-binding sites) to move independently, improving their ability to bind to multiple epitopes on an antigen simultaneously. This flexibility is particularly important for the immune system’s ability to recognize and neutralize a wide variety of pathogens. The hinge region also plays a role in the antibody’s ability to engage with other components of the immune system, such as complement proteins and immune cell receptors.
d) Define Human Leucocyte Antigen (HLA).
Answer:
iv) Human Leucocyte Antigen (HLA)
The Human Leucocyte Antigen (HLA) system refers to a group of genes located on chromosome 6 that encode the major histocompatibility complex (MHC) proteins in humans. These proteins are essential for the immune system’s ability to recognize foreign molecules and pathogens. HLA molecules present peptide fragments from antigens (either self or non-self) on the surface of cells, which can then be recognized by T cells to trigger an immune response. There are two main classes of HLA molecules: Class I (HLA-A, HLA-B, HLA-C), which present antigens to CD8+ cytotoxic T cells, and Class II (HLA-DP, HLA-DQ, HLA-DR), which present antigens to CD4+ helper T cells. The HLA system plays a critical role in immune recognition and tolerance, and variations in HLA genes can influence susceptibility to autoimmune diseases, infections, and transplant rejection.
Question:-4
a) Write a short note on Monoclonal antibodies.
Answer:
i) Monoclonal Antibodies
Monoclonal antibodies (mAbs) are laboratory-made antibodies that are derived from a single clone of a B cell, ensuring that all the antibodies are identical (monoclonal). These antibodies are highly specific to a single epitope (the part of the antigen that they bind to) and are produced using a technique called hybridoma technology. The process involves fusing a specific antibody-producing B cell with a myeloma (cancer) cell to create a hybrid cell (hybridoma) that can proliferate indefinitely and produce large quantities of the desired antibody. Monoclonal antibodies have a wide range of applications in medicine, including in cancer immunotherapy (e.g., rituximab, trastuzumab), diagnostics (e.g., pregnancy tests, detection of infectious agents), and autoimmune disease treatment (e.g., adalimumab for rheumatoid arthritis). Their precision and ability to target specific antigens make them valuable tools for both therapeutic and diagnostic purposes.
b) Write a short note on Opsonization.
Answer:
ii) Opsonization
Opsonization is the process by which pathogens, such as bacteria or viruses, are marked for destruction by the immune system. This is achieved by the binding of opsonins—antibodies (mainly IgG) or complement proteins (e.g., C3b)—to the surface of the pathogen. Opsonins enhance the ability of phagocytic cells (like macrophages, neutrophils, and dendritic cells) to recognize, engulf, and destroy the pathogen. The Fc receptors on the surface of phagocytes bind to the Fc portion of the antibody or complement fragments, promoting phagocytosis. This process is essential for the effective clearance of pathogens and the initiation of an immune response. Opsonization increases the efficiency and specificity of the immune system in fighting infections.
c) Write a short note on DNA Vaccines.
Answer:
iii) DNA Vaccines
DNA vaccines are a type of genetic vaccine that involves the direct introduction of plasmid DNA containing the genetic material for an antigen into the body. The DNA is taken up by cells, which then use the information to produce the antigen. The immune system recognizes the produced antigen as foreign and mounts an immune response. DNA vaccines can stimulate both humoral immunity (through the production of antibodies) and cell-mediated immunity (through the activation of T cells). One of the major advantages of DNA vaccines is their ability to provide strong immune protection against a variety of pathogens, including viruses and bacteria. Additionally, DNA vaccines are stable, easy to manufacture, and do not require live pathogens, which reduces safety concerns. An example of a DNA vaccine is the ZyCoV-D developed for COVID-19.
d) Write a short note on Agglutination Reactions.
Answer:
iv) Agglutination Reactions
Agglutination reactions are tests that involve the clumping (agglutination) of particles (such as bacteria, red blood cells, or latex beads) when they are exposed to specific antibodies. This occurs when the antibodies bind to antigens on the surface of the particles, cross-linking them and causing visible aggregation. Agglutination reactions are commonly used in diagnostic microbiology and immunology for detecting the presence of specific pathogens or antigens in a sample. For example, blood typing involves agglutination reactions where antibodies specific to A, B, or Rh antigens are mixed with a sample of blood to determine its blood group. Latex agglutination tests are also used to detect bacterial infections, where latex beads coated with antibodies bind to the target antigen, causing visible clumping that can be detected with the naked eye.
Question:-5
a) Differentiate between Helper " T T " cells and cytotoxic " T T " cells.
Answer:
Helper T Cells vs. Cytotoxic T Cells
1. Function and Role in the Immune System
-
Helper T Cells (CD4+ T cells):
- Role: Helper T cells are crucial for orchestrating the immune response. They do not directly kill infected cells but instead help activate and regulate other immune cells.
- Function: They assist in the activation of B cells (for antibody production), cytotoxic T cells (for killing infected cells), and macrophages (for enhanced phagocytosis). Helper T cells also promote the production of cytokines, which are signaling molecules that coordinate the immune response.
- Mechanism: Helper T cells primarily function by recognizing antigens presented by MHC class II molecules on antigen-presenting cells (APCs) like dendritic cells, macrophages, and B cells. Upon activation, they secrete cytokines that activate other immune components.
-
Cytotoxic T Cells (CD8+ T cells):
- Role: Cytotoxic T cells are responsible for identifying and destroying infected or cancerous cells. They are key players in defending the body against viral infections and tumor cells.
- Function: Cytotoxic T cells directly kill cells that display foreign antigens (such as viral or tumor antigens) on MHC class I molecules. They release cytotoxins like perforin and granzymes, which induce apoptosis (programmed cell death) in the target cell.
- Mechanism: Cytotoxic T cells recognize the foreign antigens displayed on MHC class I molecules of infected cells. Upon recognition, cytotoxic T cells release perforin, which creates pores in the target cell membrane, allowing granzymes to enter and trigger apoptosis.
2. Surface Markers and Receptors
-
Helper T Cells (CD4+ T cells):
- Helper T cells are defined by the CD4+ marker on their surface. CD4 is a co-receptor that assists in binding to MHC class II molecules on antigen-presenting cells.
-
Cytotoxic T Cells (CD8+ T cells):
- Cytotoxic T cells are defined by the CD8+ marker on their surface. CD8 is a co-receptor that assists in binding to MHC class I molecules on infected or abnormal cells.
3. Antigen Recognition
-
Helper T Cells:
- Helper T cells recognize processed antigen fragments (peptides) presented on MHC class II molecules found on antigen-presenting cells (APCs). The antigen presentation is typically derived from extracellular pathogens (such as bacteria, fungi, or parasites).
-
Cytotoxic T Cells:
- Cytotoxic T cells recognize processed antigen fragments presented on MHC class I molecules of infected or abnormal cells. These antigens are typically derived from intracellular pathogens (such as viruses) or tumor cells.
4. Activation and Cytokine Production
-
Helper T Cells:
- Upon activation, helper T cells secrete a wide array of cytokines, including interleukins (e.g., IL-2, IL-4, IL-17), which coordinate the immune response by activating other immune cells such as B cells, cytotoxic T cells, and macrophages.
- Helper T cells are divided into different subsets (Th1, Th2, Th17, Tfh, etc.), each of which produces specific cytokines to target particular immune responses.
-
Cytotoxic T Cells:
- Upon activation, cytotoxic T cells do not primarily produce cytokines. Instead, they focus on releasing cytotoxic molecules such as perforin and granzymes, which are used to kill infected cells.
- Cytotoxic T cells may also release cytokines (e.g., IFN-γ) that help recruit and activate other immune cells like macrophages.
5. Effector Mechanism
-
Helper T Cells:
- Helper T cells function as the "commanders" of the immune system, activating other immune cells to respond to pathogens.
- They do not directly engage in cell killing but instead coordinate the immune response through cytokine signaling and activation of other immune components.
-
Cytotoxic T Cells:
- Cytotoxic T cells are the "killers" in the immune response. They directly target and destroy cells infected with viruses, intracellular bacteria, or cancerous cells through the release of cytotoxins, triggering apoptosis in the target cells.
6. Role in Immune Disorders
-
Helper T Cells:
- Helper T cells are involved in both autoimmune diseases (e.g., rheumatoid arthritis, multiple sclerosis) and allergic reactions. Dysregulation of helper T cells can lead to chronic inflammation and tissue damage.
- They also play a role in HIV infection, where the virus specifically targets and destroys CD4+ T cells, weakening the immune response.
-
Cytotoxic T Cells:
- Cytotoxic T cells are essential in combating viral infections and tumors. They can become dysfunctional in certain conditions, such as autoimmunity (where they might attack healthy cells) or in the presence of immune evasion mechanisms by pathogens or cancer cells.
- Cytotoxic T cells are also implicated in graft rejection during organ transplants, where they attack transplanted tissues recognized as foreign.
7. Summary of Differences
Feature | Helper T Cells (CD4+) | Cytotoxic T Cells (CD8+) |
---|---|---|
Function | Activate and regulate other immune cells | Directly kill infected or cancerous cells |
Antigen Presentation | MHC Class II molecules on APCs | MHC Class I molecules on infected cells |
Surface Marker | CD4 | CD8 |
Effector Mechanism | Secrete cytokines, activate B cells and macrophages | Release perforin and granzymes to kill target cells |
Main Role | Coordinate immune responses | Kill infected or tumor cells |
Cytokine Production | Yes, various cytokines (e.g., IL-2, IL-4, IL-17) | Yes, but mainly for killing cells (e.g., IFN-γ) |
In conclusion, Helper T cells are essential for orchestrating the immune response by activating other immune cells, whereas Cytotoxic T cells specialize in directly killing infected or abnormal cells, making each of these T cell subsets crucial for specific aspects of immune defense.
b) Differentiate between Active and Passive immunity.
Answer:
Active Immunity vs. Passive Immunity
1. Definition
-
Active Immunity: Active immunity occurs when an individual’s immune system is exposed to a pathogen or its antigens and subsequently produces an immune response, including the production of antibodies and memory cells. This type of immunity is typically long-lasting, as it involves the body’s own immune system developing a defense.
-
Passive Immunity: Passive immunity refers to the transfer of pre-formed antibodies or immune cells from another individual or organism, providing immediate but temporary protection. The recipient’s immune system does not actively participate in generating the immune response.
2. Mechanism of Immunity
-
Active Immunity:
- Process: In active immunity, exposure to a pathogen (via infection or vaccination) stimulates the immune system to produce antibodies and activate T cells. The immune system also generates memory cells (B cells and T cells), which "remember" the pathogen, leading to a quicker and more effective response upon future exposures.
- Response: The body’s immune system actively produces antibodies and induces a cell-mediated response (including helper T cells and cytotoxic T cells) to fight the infection or disease.
-
Passive Immunity:
- Process: Passive immunity involves the transfer of antibodies from an external source. These antibodies can be acquired naturally (e.g., through the placenta or breast milk) or artificially (e.g., through antibody-containing blood products such as immune globulin).
- Response: The recipient’s immune system does not generate any new antibodies or immune memory; it only benefits from the antibodies provided by another source.
3. Duration of Immunity
-
Active Immunity:
- Duration: Active immunity is generally long-lasting, often providing years or even lifetime protection. This is because memory cells persist in the body, allowing the immune system to mount a rapid and effective response if the pathogen is encountered again.
-
Passive Immunity:
- Duration: Passive immunity provides temporary protection, usually lasting only a few weeks to a few months. This is because the transferred antibodies eventually degrade over time, and no memory cells are formed in the recipient’s immune system.
4. Examples
-
Active Immunity:
- Vaccination: Vaccines, such as those for measles, influenza, or COVID-19, stimulate the body to produce antibodies and memory cells without causing the disease itself.
- Natural Infection: After recovery from an infection (e.g., chickenpox or influenza), the immune system has developed immunity against future infections by the same pathogen.
-
Passive Immunity:
- Natural: Maternal antibodies passed from mother to fetus through the placenta during pregnancy, or through breast milk, provide temporary protection to the infant against infections during the early months of life.
- Artificial: The administration of immunoglobulins (antibody-rich blood products) to individuals who have been exposed to specific pathogens, such as in cases of tetanus, rabies, or hepatitis B exposure, to provide immediate protection.
5. Immunological Memory
-
Active Immunity:
- Memory Formation: Active immunity involves the creation of memory B and T cells. These cells remain in the body long after the infection or vaccination, enabling the immune system to respond more rapidly and effectively if the pathogen is encountered again.
-
Passive Immunity:
- No Memory Formation: In passive immunity, the immune system does not create memory cells, meaning that there is no long-term immune defense once the transferred antibodies degrade.
6. Speed of Protection
-
Active Immunity:
- Onset of Protection: Active immunity takes time to develop. After exposure to the pathogen or vaccination, it typically takes several days to weeks for the immune system to generate an effective response, including the production of antibodies and the activation of memory cells.
-
Passive Immunity:
- Onset of Protection: Passive immunity provides immediate protection since the antibodies are already present in the recipient. This makes it useful in situations where immediate defense against infection is needed, such as after exposure to certain viruses or bacteria.
7. Advantages and Disadvantages
-
Active Immunity:
- Advantages: Long-lasting protection, development of immune memory, and no need for repeated treatments.
- Disadvantages: Takes time to develop protection, and there is a risk of side effects (e.g., mild symptoms from vaccines or illness from natural infection).
-
Passive Immunity:
- Advantages: Provides immediate protection, ideal in emergency situations or for individuals who cannot mount their own immune response (e.g., immunocompromised patients).
- Disadvantages: Temporary protection with no long-term immunity, and the potential for allergic reactions to the foreign antibodies.
8. Summary Table
Feature | Active Immunity | Passive Immunity |
---|---|---|
Mechanism | Immune system actively produces antibodies and memory cells | Transfer of pre-formed antibodies from another source |
Duration | Long-lasting (years or lifetime) | Short-term (weeks to months) |
Examples | Vaccination, natural infection | Maternal antibodies, antibody treatments (e.g., immunoglobulins) |
Memory Formation | Yes, memory B and T cells | No memory formation |
Speed of Protection | Slow (takes time to develop immune response) | Immediate protection |
Protection Type | Adaptive immunity (active involvement of the immune system) | Passive immunity (no immune system involvement) |
Advantages | Long-term protection, memory cells, no need for re-treatment | Immediate protection, useful in emergencies |
Disadvantages | Delayed onset of protection, potential side effects | Short-lived immunity, no long-term defense |
Conclusion
In summary, active immunity is a self-generated immune response involving the production of antibodies and memory cells, providing long-lasting protection against future infections. It is typically acquired through infection or vaccination. On the other hand, passive immunity involves the transfer of pre-formed antibodies from another source, offering immediate but temporary protection, without the formation of memory cells. While both types of immunity play important roles in the body’s defense against disease, active immunity provides durable protection, while passive immunity offers quick, short-term defense.
c) Differentiate between MHC class-I and II molecules.
Answer:
Differences Between MHC Class I and Class II Molecules
1. Structure and Composition
-
MHC Class I Molecules:
- MHC class I molecules consist of a single alpha chain and a beta-2 microglobulin (β2m). The alpha chain is divided into three domains (α1, α2, and α3), with the α1 and α2 domains forming a groove that holds the antigenic peptide. The α3 domain binds to CD8 co-receptors on cytotoxic T cells.
-
MHC Class II Molecules:
- MHC class II molecules are composed of two polypeptide chains: an alpha chain and a beta chain. Both chains are integral to forming the antigen-binding groove, which holds the antigenic peptide. The CD4 co-receptor on helper T cells binds to the β2 domain of MHC class II molecules.
2. Expression and Distribution
-
MHC Class I Molecules:
- MHC class I molecules are expressed on the surface of all nucleated cells, including epithelial cells, muscle cells, and neurons. These molecules are crucial for displaying intracellular antigens such as those from viruses or tumors.
-
MHC Class II Molecules:
- MHC class II molecules are predominantly expressed on antigen-presenting cells (APCs), including dendritic cells, macrophages, and B cells. These cells are specialized in capturing, processing, and presenting extracellular antigens.
3. Type of Antigen Presented
-
MHC Class I Molecules:
- MHC class I molecules primarily present endogenous antigens, which are peptides derived from proteins synthesized inside the cell. These proteins can be viral proteins, mutated tumor proteins, or self-proteins that are abnormal. The presented peptides are typically 8-10 amino acids in length.
-
MHC Class II Molecules:
- MHC class II molecules present exogenous antigens derived from extracellular sources, such as bacteria, fungi, and parasites. These antigens are taken up by the APCs through phagocytosis or endocytosis, processed, and displayed. The peptides are usually longer (13-25 amino acids in length).
4. Interaction with T Cells
-
MHC Class I Molecules:
- MHC class I molecules interact with CD8+ cytotoxic T cells (also known as killer T cells). The CD8 co-receptor on cytotoxic T cells binds to the α3 domain of the MHC class I molecule, facilitating the recognition and killing of infected or tumor cells that present foreign peptides.
-
MHC Class II Molecules:
- MHC class II molecules interact with CD4+ helper T cells. The CD4 co-receptor on helper T cells binds to the β2 domain of the MHC class II molecule. This interaction helps activate helper T cells, which in turn stimulate B cells to produce antibodies and macrophages to phagocytize pathogens.
5. Antigen Processing Pathway
-
MHC Class I Molecules:
- The antigen processing pathway for MHC class I involves the cytosolic degradation of proteins by the proteasome. These degraded peptides are transported into the endoplasmic reticulum (ER) by the TAP (Transporter Associated with Antigen Processing) complex, where they bind to MHC class I molecules. The peptide-MHC class I complex is then transported to the cell surface via the Golgi apparatus.
-
MHC Class II Molecules:
- MHC class II molecules are processed in endosomal compartments within antigen-presenting cells. The antigens are taken up from the extracellular environment, degraded in phagosomes or endosomes, and the peptides are then loaded onto the MHC class II molecules. The peptide-MHC class II complex is subsequently transported to the cell surface for presentation to CD4+ T cells.
6. Role in Immune Response
-
MHC Class I Molecules:
- The primary role of MHC class I molecules is to present antigens to cytotoxic T cells (CD8+) for the detection and destruction of infected or cancerous cells. This is crucial for the immune system’s response to viruses, intracellular pathogens, and tumors.
-
MHC Class II Molecules:
- MHC class II molecules are involved in the activation of helper T cells (CD4+). Once activated, helper T cells assist in the immune response by stimulating other immune cells, including B cells (for antibody production) and macrophages (for enhanced pathogen clearance), to fight off extracellular pathogens like bacteria and fungi.
7. Genetic Differences and Polymorphism
-
MHC Class I Molecules:
- The genes encoding MHC class I molecules are part of the human leukocyte antigen (HLA) complex, specifically HLA-A, HLA-B, and HLA-C loci. These molecules are highly polymorphic, meaning they have many different versions (alleles) within the population, which enhances the ability of the immune system to recognize a wide variety of pathogens.
-
MHC Class II Molecules:
- MHC class II molecules are encoded by the HLA-D region of the genome, which includes HLA-DP, HLA-DQ, and HLA-DR genes. Like MHC class I, MHC class II molecules are also polymorphic, allowing for a broad range of antigen presentation.
8. Summary Table
Feature | MHC Class I Molecules | MHC Class II Molecules |
---|---|---|
Structure | Single alpha chain + beta-2 microglobulin | Alpha chain + beta chain |
Expression | All nucleated cells | Antigen-presenting cells (dendritic cells, macrophages, B cells) |
Antigen Type | Endogenous (intracellular) antigens | Exogenous (extracellular) antigens |
T Cell Interaction | Interacts with CD8+ cytotoxic T cells | Interacts with CD4+ helper T cells |
Antigen Processing Pathway | Proteasome degradation → TAP transport → ER → surface presentation | Phagocytosis/endocytosis → lysosomal degradation → surface presentation |
Role | Detection of infected or tumor cells, activation of cytotoxic T cells | Activation of helper T cells, immune coordination |
Genetic Loci | HLA-A, HLA-B, HLA-C | HLA-DP, HLA-DQ, HLA-DR |
Conclusion
In summary, MHC class I molecules are critical for presenting endogenous antigens to CD8+ cytotoxic T cells, primarily involved in defending against intracellular infections and tumors. In contrast, MHC class II molecules present exogenous antigens to CD4+ helper T cells, which are essential for initiating broader immune responses to extracellular pathogens. Both classes of molecules are highly polymorphic, allowing the immune system to effectively recognize a diverse range of pathogens.
d) Differentiate between Self and non-self antigens.
Answer:
Differences Between Self and Non-Self Antigens
1. Definition
-
Self Antigens:
- Self antigens are molecules or markers that are naturally present on the cells and tissues of an individual’s own body. These molecules are recognized as "self" by the immune system and are typically tolerated by the immune system, meaning the body does not mount an immune response against them under normal circumstances.
-
Non-Self Antigens:
- Non-self antigens are molecules or markers that originate from external sources such as pathogens (viruses, bacteria, fungi, parasites), foreign cells (e.g., from organ transplants), or environmental agents. The immune system recognizes these as "foreign" and mounts an immune response to eliminate them.
2. Origin
-
Self Antigens:
- Self antigens are produced by the body itself, including proteins, lipids, and other molecules that are naturally expressed by the cells and tissues of an individual.
-
Non-Self Antigens:
- Non-self antigens are derived from external sources, such as microbes (bacteria, viruses, etc.), allergens (e.g., pollen or dust), or even foreign tissues (e.g., during organ transplantation).
3. Recognition by the Immune System
-
Self Antigens:
- The immune system typically does not react to self antigens because the body has mechanisms for recognizing its own molecules. This recognition involves immune tolerance, which prevents the immune system from attacking its own cells and tissues.
-
Non-Self Antigens:
- The immune system recognizes non-self antigens as foreign and dangerous, initiating an immune response to neutralize or eliminate the threat. This involves the activation of various immune components, such as T cells, B cells, and antibodies.
4. Immune Response
-
Self Antigens:
- Under normal, healthy conditions, self antigens do not induce an immune response. However, if the immune system fails to recognize self antigens due to immune dysregulation or malfunction, it may result in autoimmune diseases where the body attacks its own tissues (e.g., rheumatoid arthritis, type 1 diabetes, multiple sclerosis).
-
Non-Self Antigens:
- Non-self antigens trigger an immune response, which typically involves the production of antibodies, activation of cytotoxic T cells, and other immune cells designed to neutralize or eliminate the foreign antigen. This response is crucial for fighting infections and preventing the spread of disease.
5. Examples
-
Self Antigens:
- Major Histocompatibility Complex (MHC) molecules on the surface of cells, which help the immune system recognize "self."
- Blood group antigens (e.g., ABO antigens).
- Self proteins in tissues like collagen or enzymes involved in metabolism.
-
Non-Self Antigens:
- Pathogen-derived antigens: such as viral proteins (e.g., influenza hemagglutinin), bacterial toxins (e.g., tetanus toxin), and fungal components.
- Transplanted organ cells or tissue, which express foreign antigens not recognized by the recipient’s immune system.
- Allergens: environmental substances like pollen, dust mites, or pet dander that trigger allergic reactions.
6. Role in Autoimmunity
-
Self Antigens:
- In autoimmune diseases, the immune system mistakenly recognizes self antigens as foreign, leading to an attack on its own tissues. For example, in Systemic Lupus Erythematosus (SLE), the immune system attacks the body’s own DNA, and in Hashimoto’s thyroiditis, the immune system targets the thyroid gland.
-
Non-Self Antigens:
- Non-self antigens are normally targeted by the immune system in a protective response. However, in hypersensitivity reactions, the immune system may overreact to non-harmful non-self antigens (e.g., pollen or peanuts), resulting in allergic reactions.
7. Tolerance Mechanisms
-
Self Antigens:
- The immune system develops tolerance to self antigens through processes such as central tolerance (where self-reactive T and B cells are eliminated in the thymus and bone marrow) and peripheral tolerance (where self-reactive cells are inactivated or suppressed in peripheral tissues). These mechanisms prevent the immune system from attacking the body’s own cells.
-
Non-Self Antigens:
- The immune system does not develop tolerance to non-self antigens; rather, it actively recognizes and targets them as foreign. This recognition triggers a cascade of immune responses aimed at neutralizing or removing the foreign invader.
8. Summary Table
Feature | Self Antigens | Non-Self Antigens |
---|---|---|
Origin | Produced by the body itself | Derived from external sources (pathogens, allergens, etc.) |
Recognition by the Immune System | Immune system recognizes as "self," no immune response under normal conditions | Immune system recognizes as "foreign" and mounts a response |
Immune Response | None, unless in autoimmune diseases | Active immune response (e.g., antibodies, T cell activation) |
Examples | MHC molecules, blood group antigens, self proteins | Pathogen antigens, allergens, transplanted tissue |
Role in Autoimmunity | Can cause autoimmune diseases if tolerance is broken | Not involved in autoimmunity, typically causes immune defense |
Tolerance Mechanisms | Central and peripheral tolerance prevent immune response | No tolerance mechanisms, immune system targets foreign antigens |
Conclusion
In summary, self antigens are naturally occurring molecules within the body that the immune system recognizes as "self" and does not typically mount an immune response against. However, when this self-recognition fails, autoimmune diseases can occur. Non-self antigens, on the other hand, originate from external sources and are recognized as foreign by the immune system, prompting an immune response to eliminate the perceived threat. The immune system’s ability to distinguish between self and non-self is crucial for maintaining health and preventing both infections and autoimmune conditions.
Question:-6
What is the vaccine? Explain the mode of action of vaccine. Write about the advantages and disadvantages of vaccination.
Answer:
1. Introduction to Vaccines
A vaccine is a biological preparation that provides acquired immunity to a particular infectious disease. It typically contains an agent that resembles a disease-causing microorganism and is often made from weakened or killed forms of the microbe, its toxins, or one of its surface proteins. Vaccines stimulate the immune system to recognize and fight pathogens, making it more efficient at recognizing and neutralizing future exposures to the same pathogen.
Vaccination is considered one of the most effective public health strategies for preventing the spread of infectious diseases, including diseases like polio, measles, influenza, and hepatitis B.
2. Mode of Action of Vaccines
Vaccines work by mimicking an infection, but without causing the actual disease. This process helps the immune system prepare itself for future encounters with the pathogen. The mode of action can be broken down into several steps:
-
Exposure to Antigen: When a person is vaccinated, they are exposed to a harmless part of the pathogen, known as an antigen. This can be a whole microorganism (such as a virus or bacterium), a piece of the microorganism (such as a protein or sugar), or a toxin that the microorganism produces.
-
Activation of Immune Response: The immune system recognizes the antigen as foreign and responds by activating the innate immune system. This includes the production of phagocytes, which engulf the foreign particles. The adaptive immune system is then triggered, involving T cells (which coordinate the immune response) and B cells (which produce antibodies).
-
Production of Antibodies: B cells, once activated, produce specific antibodies tailored to recognize and neutralize the pathogen. These antibodies remain in circulation for a period of time, offering passive immunity.
-
Formation of Memory Cells: After the immune system successfully responds to the pathogen, it forms memory cells—both memory B cells and memory T cells. These cells “remember” the pathogen and remain in the body for years or even a lifetime. In case of future exposure, the immune system can rapidly recognize and attack the pathogen, often preventing illness from occurring.
-
Neutralization of Future Infection: Upon subsequent exposure to the same pathogen, the immune system is able to quickly respond due to the presence of the memory cells, leading to a much faster and more efficient immune response. This rapid response often prevents the disease from taking hold, or at least significantly reduces its severity.
3. Types of Vaccines
Vaccines come in different forms, and they can be classified based on the components of the pathogen they contain:
-
Inactivated or Killed Vaccines: These vaccines contain pathogens that have been killed or inactivated so they cannot cause disease. Examples include the polio vaccine (IPV) and the hepatitis A vaccine.
-
Live Attenuated Vaccines: These vaccines contain live pathogens that have been weakened so that they cannot cause illness in healthy individuals. They closely mimic a natural infection and produce a strong and lasting immune response. Examples include the measles, mumps, rubella (MMR) vaccine and the yellow fever vaccine.
-
Subunit, Recombinant, or Conjugate Vaccines: These vaccines contain pieces of the pathogen, such as proteins or sugars, that trigger an immune response without containing the entire pathogen. Examples include the Hepatitis B vaccine and the Haemophilus influenzae type b (Hib) vaccine.
-
Toxoid Vaccines: These vaccines are made from inactivated toxins produced by the pathogen. They help protect against diseases caused by bacterial toxins. Examples include the diphtheria and tetanus vaccines.
4. Advantages of Vaccination
Vaccination has numerous benefits for individuals and public health:
-
Prevention of Disease: Vaccines prevent a variety of diseases, many of which can cause severe illness or death. For example, the measles vaccine prevents measles, which can lead to complications like pneumonia, brain swelling, and death.
-
Herd Immunity: When a significant portion of the population is vaccinated, it creates herd immunity, which makes it difficult for a disease to spread. This protects individuals who cannot be vaccinated, such as those with weakened immune systems (e.g., chemotherapy patients or infants who are too young for certain vaccines).
-
Eradication of Diseases: Vaccines have contributed to the eradication of diseases like smallpox and the near-eradication of polio. Through widespread vaccination, the goal is to completely eliminate the disease from the population.
-
Cost-Effective: Vaccination is a cost-effective way to prevent diseases compared to treating the disease once it occurs. It helps reduce the financial burden on healthcare systems by avoiding hospitalizations, long-term treatments, and lost productivity.
-
Reduction of Disease Spread: By preventing the spread of infectious diseases, vaccination helps reduce the burden on healthcare systems and decreases the overall prevalence of diseases in the community.
-
Protection Against Emerging Threats: Vaccines are developed in response to emerging infectious diseases, as seen with the rapid development of the COVID-19 vaccines. This allows for a quick response to new pandemics.
5. Disadvantages of Vaccination
While vaccines are highly beneficial, there are some disadvantages or limitations to consider:
-
Side Effects and Reactions: Most vaccines have mild side effects, such as fever, pain at the injection site, or fatigue. Rarely, vaccines may cause more severe reactions, such as allergic responses. However, these reactions are generally much less common than the adverse effects caused by the diseases the vaccines prevent.
-
Incomplete Protection: While vaccines provide protection against many diseases, they may not provide 100% immunity. Some individuals may not develop full immunity after vaccination, and they may remain vulnerable to the disease. For example, the influenza vaccine is not always perfectly matched to circulating strains, which can reduce its effectiveness in some years.
-
Vaccine Hesitancy: There is a growing issue of vaccine hesitancy in certain populations, driven by misinformation, fear, and distrust in medical institutions. This can lead to lower vaccination rates and outbreaks of vaccine-preventable diseases.
-
Access and Availability: Vaccines may not be readily available or accessible in all regions, especially in low-income countries or areas with poor healthcare infrastructure. This can limit the effectiveness of global vaccination efforts, leaving some populations vulnerable to preventable diseases.
-
Cost of Vaccines: While vaccines are generally cost-effective in the long run, the initial development, production, and distribution costs can be high. This may create financial barriers to widespread vaccination, particularly in developing countries.
-
Potential for Misuse: There is the potential for vaccines to be used improperly, for example, when vaccine schedules are not followed, or vaccines are not stored properly, reducing their efficacy.
6. Conclusion
Vaccination plays a critical role in modern medicine by preventing the spread of infectious diseases, saving lives, and promoting public health. The mode of action involves stimulating the immune system to recognize and fight specific pathogens without causing the disease itself, and the benefits include the prevention of serious diseases, the promotion of herd immunity, and the eradication of certain diseases. While vaccines are generally safe and highly effective, they come with some limitations, including side effects, incomplete protection, and issues related to access and vaccine hesitancy. Despite these drawbacks, the advantages of vaccination far outweigh the disadvantages, making it one of the most important tools in global health.
Question:-7
a) Write the various steps in the establishment of bacterial infection.
Answer:
1. Introduction to Bacterial Infection
A bacterial infection occurs when harmful bacteria enter the body and begin to proliferate, potentially causing damage to tissues and triggering an immune response. The establishment of bacterial infections is a complex process involving multiple stages. Understanding how bacteria invade and infect host tissues is essential for developing effective treatments and preventive measures. The process of bacterial infection typically involves a series of steps, from the initial encounter with the host to the establishment of an active infection.
2. Encounter and Entry into the Host
The first step in the establishment of a bacterial infection is the encounter between the bacterium and the host. This usually happens through various routes of exposure, such as:
- Respiratory tract: Bacteria can be inhaled through airborne droplets (e.g., in pneumonia or tuberculosis).
- Gastrointestinal tract: Ingested contaminated food or water (e.g., in foodborne infections like Salmonella or E. coli).
- Skin or mucous membranes: Direct contact with contaminated surfaces or injury (e.g., in wound infections, cellulitis).
- Genitourinary tract: Bacteria can enter through sexual contact or improper hygiene (e.g., in urinary tract infections).
Once bacteria come into contact with the host, they need to breach the body’s initial defenses, such as the skin, mucosal barriers, or epithelial cells.
3. Adherence to Host Cells
After successfully entering the host, bacteria must adhere to host tissues to establish a foothold. This process is mediated by various adhesion factors such as:
- Pili (fimbriae): Hair-like structures on bacterial surfaces that allow attachment to host cell receptors.
- Adhesins: Surface proteins that bind to specific molecules on host cells, facilitating attachment.
- Capsules: Some bacteria have a slimy outer coating that helps them adhere to surfaces, as well as evade immune detection.
Adherence is crucial because it allows bacteria to resist being flushed out by physical barriers like mucus, saliva, or the flow of urine. The stronger the adhesion, the more likely the bacteria are to establish an infection.
4. Colonization and Growth
Once bacteria have adhered to host tissues, they begin colonizing the area. This involves multiplying and forming a local population. Bacteria can thrive in specific environments depending on their characteristics. For example, some bacteria grow best in oxygen-rich environments (aerobic bacteria), while others may require low-oxygen conditions (anaerobic bacteria).
Bacterial colonization often involves the production of biofilms, which are clusters of bacteria embedded in a matrix of their own secreted material. Biofilms help bacteria to adhere more strongly to surfaces, protect themselves from the immune system, and resist antibiotic treatments.
During this phase, bacteria utilize nutrients from the host (like iron, sugars, and amino acids) to fuel their growth and multiplication. This step is critical for the infection to become established as a significant, active infection.
5. Invasion of Host Tissues
In many infections, bacteria do not remain confined to the surface of tissues but must invade deeper into the host. This can involve the production of enzymes and other factors that allow the bacteria to penetrate host cell membranes and extracellular matrix components. These factors may include:
- Proteases: Enzymes that break down proteins and allow bacteria to penetrate tissue barriers.
- Hyaluronidase: An enzyme that breaks down the hyaluronic acid in connective tissues, enabling bacterial spread.
- Collagenase: An enzyme that degrades collagen, a major structural component of connective tissues.
In some cases, bacteria can enter host cells directly through endocytosis, where the host cell engulfs the bacteria into vacuoles.
Once inside the host’s tissues, bacteria can spread through the bloodstream (bacteremia) or lymphatic system, potentially causing systemic infection and spreading to distant organs.
6. Evasion of Host Immune System
As bacteria establish an infection, they must overcome the host’s immune defenses, which include both innate and adaptive immunity. To evade immune detection, bacteria employ various strategies:
- Capsules: Some bacteria produce capsules that prevent phagocytosis by immune cells like macrophages and neutrophils.
- Antigenic variation: Bacteria can change their surface proteins to avoid recognition by the host’s antibodies.
- Toxins: Many bacteria secrete toxins that damage immune cells or suppress immune responses. For example, staphylococcal enterotoxins can interfere with the functioning of immune cells.
- Immune suppression: Some bacteria, like Mycobacterium tuberculosis, can survive within host cells, preventing the host immune system from effectively targeting them.
The ability to evade the immune response is a key factor in the persistence of infection and in the severity of disease.
7. Damage to Host Tissues
Bacterial infections often lead to tissue damage either directly through the action of bacterial toxins or indirectly by triggering an immune response. Bacteria can produce a variety of toxins that contribute to tissue damage:
- Exotoxins: These are toxic proteins secreted by bacteria into the host’s tissues. Examples include diphtheria toxin and botulinum toxin.
- Endotoxins: Found in the outer membrane of Gram-negative bacteria, these toxins can trigger severe inflammatory responses when released into the bloodstream, leading to conditions like sepsis.
The host’s immune system also contributes to tissue damage through the production of inflammatory molecules like cytokines, which recruit immune cells to the site of infection but can also cause collateral damage to host tissues.
8. Resolution or Chronic Infection
In the final stages of bacterial infection, the host’s immune system may either resolve the infection or allow it to become chronic:
-
Resolution: If the immune response is successful, the bacteria are cleared, and the infection resolves. This often leads to the development of immunity, as memory cells are formed to recognize the pathogen in future encounters.
-
Chronic Infection: In some cases, the bacteria evade immune clearance or overwhelm the immune system, leading to a chronic infection. Chronic infections can result in persistent inflammation, tissue damage, and long-term health problems. Examples include chronic urinary tract infections or chronic tuberculosis.
9. Conclusion
The establishment of bacterial infection involves a series of complex steps, including the entry of bacteria into the host, adherence to host tissues, colonization, invasion of deeper tissues, evasion of the immune system, and eventual tissue damage. Successful bacterial infections depend on the pathogen’s ability to overcome the host’s defenses and utilize host resources for growth. These infections can range from acute, self-limiting diseases to chronic, persistent conditions. Understanding the steps involved in bacterial infection provides valuable insights into how infections progress and how we can develop strategies to treat or prevent them.
b) Explain the neutralization mechanism of bacteria by antibodies.
Answer:
1. Introduction to Neutralization Mechanism
Neutralization is one of the key ways antibodies help to protect the host from bacterial infections. It refers to the process by which antibodies bind to bacterial pathogens or their toxins and inhibit their ability to cause harm. This mechanism is crucial in the immune system’s response to infection, as it prevents bacteria from adhering to and invading host cells, neutralizes harmful toxins, and facilitates their clearance from the body. Antibodies are produced by B cells in response to bacterial infections and are essential for both humoral immunity and the overall immune defense.
2. Structure of Antibodies Involved in Neutralization
Antibodies, also known as immunoglobulins (Ig), are glycoproteins that have a specific structure that allows them to recognize and bind to particular antigens (molecules on pathogens such as bacteria or their toxins). The structure of an antibody consists of two main parts:
- Variable Region: This region of the antibody is responsible for binding to the specific antigen. It is highly variable between different antibodies, allowing the immune system to recognize a vast array of pathogens.
- Constant Region: This part of the antibody remains the same across different antibodies of the same class. The constant region interacts with other components of the immune system, such as phagocytes and complement proteins, to help clear the pathogen.
The Fab region (antigen-binding fragment) binds to the antigen, while the Fc region (crystallizable fragment) interacts with immune cells to trigger responses like phagocytosis.
3. Antibody Binding to Bacterial Pathogens
The first step in the neutralization of bacteria involves antibodies binding to the bacterial surface antigens. These antigens could be proteins, lipids, or polysaccharides present on the bacterial cell surface. By binding to these antigens, antibodies can block several key processes necessary for bacterial infection:
-
Inhibition of Adhesion: Many bacteria cause infection by adhering to host cells. Antibodies can block adhesion molecules on the bacterial surface, preventing the bacteria from attaching to host tissues and gaining access to the cells.
-
Blocking Receptors: Some bacteria have receptors that allow them to interact with host cells. Antibodies can block these receptors, preventing the bacteria from entering the cells and causing infection.
-
Inhibition of Toxin Activity: Some bacteria release toxins that damage tissues or interfere with cellular functions. Antibodies can bind to these toxins, neutralizing their harmful effects. For example, the diphtheria toxin can be neutralized by antibodies, rendering it incapable of interfering with host cell functions.
4. Neutralization of Bacterial Toxins
Bacterial toxins are often responsible for the severity of infections. These toxins can have a wide variety of effects, including disrupting cellular functions, inducing inflammation, and causing tissue damage. Antibodies can neutralize toxins in the following ways:
-
Direct Binding to Toxins: Antibodies can bind to the active sites of bacterial toxins, preventing them from interacting with host cells. For example, antibodies to botulinum toxin bind to the toxin and prevent it from binding to the neuromuscular junction, thereby preventing paralysis.
-
Blocking Toxin Receptors: Some toxins need to bind to specific receptors on the surface of host cells to be effective. By blocking the receptor binding site, antibodies prevent the toxin from interacting with its target cells, neutralizing its effect.
-
Preventing Toxin Internalization: Many bacterial toxins enter host cells by being endocytosed into vesicles. Antibodies can prevent this internalization process by binding to the toxin and blocking the necessary receptors involved in this uptake.
5. Role of Complement Activation in Neutralization
Once antibodies bind to bacterial pathogens, they can activate the complement system, a group of proteins that play a key role in the immune response. The complement system can enhance the neutralization process in several ways:
-
Opsonization: Antibodies that bind to bacteria act as "tags" that signal for phagocytes (like macrophages and neutrophils) to engulf and destroy the bacteria. This process is known as opsonization. The Fc region of the antibody interacts with complement proteins and receptors on phagocytes, facilitating the clearance of the bacteria.
-
Membrane Attack Complex: The complement system can be activated by the classical pathway, where antibodies bound to the pathogen initiate a cascade of complement activation. This leads to the formation of a membrane attack complex (MAC), which can create pores in the bacterial cell membrane, leading to bacterial lysis and death.
-
Enhancement of Antibody Functions: The complement system can also enhance the ability of antibodies to neutralize pathogens by making the pathogens more "visible" to the immune system.
6. Immune Complex Formation and Removal
In the neutralization process, when antibodies bind to bacterial antigens, they can form immune complexes. These complexes can aid in neutralization in several ways:
-
Immune Complexes: The binding of multiple antibodies to antigens on the bacterial surface leads to the formation of immune complexes, which can be cleared from the bloodstream by macrophages and other phagocytic cells.
-
Precipitation: In some cases, the immune complexes formed by antibodies and bacterial antigens may precipitate (settle out of solution), which also helps in their removal from the host body.
-
Phagocytosis: Immune complexes make bacteria more prone to being ingested and destroyed by phagocytes. The Fc region of the antibody facilitates recognition and ingestion by phagocytes through interactions with receptors on these immune cells.
7. Memory Response and Long-term Immunity
Neutralization by antibodies not only protects the body during an initial infection but also contributes to long-term immunity. Following the resolution of an infection, memory B cells are produced that “remember” the pathogen. Upon re-exposure to the same pathogen, these memory B cells quickly produce the same antibodies, enabling the immune system to neutralize the pathogen more effectively and rapidly. This process is a cornerstone of adaptive immunity and is the basis for the effectiveness of vaccines.
8. Conclusion
The neutralization of bacterial pathogens by antibodies is a fundamental mechanism of the immune response that prevents infection and tissue damage. By binding to bacteria, their toxins, and surface antigens, antibodies can block their ability to infect host cells and neutralize harmful substances. This process is further supported by the activation of the complement system, the formation of immune complexes, and the clearance of pathogens by phagocytic cells. Ultimately, antibodies contribute to both immediate protection and long-lasting immunity, providing the host with a defense against future infections by the same pathogen.
Question:-8
a) Define autoimmunity. Describe any two autoimmune disorders.
Answer:
1. Introduction to Autoimmunity
Autoimmunity refers to a condition in which the body’s immune system mistakenly attacks its own cells, tissues, and organs. The immune system, which is designed to protect the body from foreign invaders such as bacteria, viruses, and other pathogens, becomes dysregulated. In autoimmune diseases, the immune system fails to differentiate between self and non-self, targeting healthy cells as if they were harmful. This can lead to inflammation, tissue damage, and a range of symptoms that vary depending on the specific autoimmune disorder.
Autoimmunity can be triggered by various factors, including genetic predisposition, infections, environmental influences, and hormonal changes. While the exact mechanisms are not fully understood, autoimmune diseases can range from mild to life-threatening, and they often require long-term management.
2. Mechanisms Behind Autoimmunity
The immune system operates through two main components: the innate immune system (which provides a first line of defense) and the adaptive immune system (which provides a more specific response to pathogens). In autoimmunity, the adaptive immune system becomes improperly activated.
The key elements involved in autoimmunity include:
- Loss of Immune Tolerance: The immune system usually has mechanisms that prevent it from attacking the body’s own tissues. This is known as self-tolerance. In autoimmune diseases, these mechanisms fail, and immune cells that normally ignore self-antigens begin to attack them.
- Autoantibodies: In many autoimmune disorders, the immune system produces antibodies that target self-antigens. These antibodies are referred to as autoantibodies. They can bind to healthy tissues and cause inflammation and tissue damage.
- T-cell Dysregulation: T-cells play a critical role in the immune system. In autoimmune diseases, autoreactive T-cells can attack self-tissues, leading to immune-mediated damage.
The causes of autoimmunity are multifactorial and involve both genetic and environmental factors. In many cases, the environmental triggers, such as infections or exposure to certain chemicals, can initiate the development of autoimmune diseases in genetically susceptible individuals.
3. Systemic Lupus Erythematosus (SLE)
Systemic lupus erythematosus (SLE) is one of the most well-known autoimmune diseases. It is a chronic inflammatory disease that can affect almost any organ system, including the skin, joints, kidneys, heart, lungs, and nervous system. SLE is characterized by the presence of autoantibodies, particularly antinuclear antibodies (ANA), which target the nucleus of cells in the body.
-
Symptoms: The symptoms of SLE can be highly variable and can range from mild to severe. Common symptoms include:
- Butterfly-shaped rash across the cheeks and nose.
- Fatigue, joint pain, and muscle aches.
- Photosensitivity (sensitivity to sunlight).
- Kidney damage (lupus nephritis) in some cases.
- Raynaud’s phenomenon (reduced blood flow to fingers and toes).
- Chest pain, headaches, and cognitive issues.
-
Pathogenesis: The pathogenesis of SLE involves the formation of immune complexes (antibody-antigen complexes) that deposit in various tissues, triggering an inflammatory response. These immune complexes activate complement proteins, leading to inflammation, tissue damage, and the symptoms of the disease. The kidneys are particularly vulnerable, and untreated lupus can lead to renal failure.
-
Diagnosis: The diagnosis of SLE is based on clinical symptoms, laboratory findings (including the presence of ANA and anti-dsDNA antibodies), and organ involvement. Imaging studies and biopsy may be required to assess the extent of organ damage.
-
Treatment: There is no cure for SLE, but treatments focus on controlling symptoms, reducing inflammation, and preventing flares. Nonsteroidal anti-inflammatory drugs (NSAIDs), antimalarials (like hydroxychloroquine), and immunosuppressive drugs (like corticosteroids and methotrexate) are commonly used. In severe cases, biologic therapies targeting specific immune pathways (such as B-cell depletion therapies) may be recommended.
4. Rheumatoid Arthritis (RA)
Rheumatoid arthritis (RA) is another common autoimmune disease that primarily affects the joints but can also involve other organ systems. RA is a chronic inflammatory disorder that causes pain, swelling, and stiffness in the joints, particularly in the hands, wrists, knees, and feet. It is an example of a systemic autoimmune disorder, meaning it can affect tissues throughout the body.
-
Symptoms: The hallmark symptoms of RA include:
- Joint pain and swelling, particularly in the smaller joints (e.g., fingers, wrists).
- Morning stiffness, lasting for at least 30 minutes.
- Fatigue, low-grade fever, and weight loss.
- In some cases, rheumatoid nodules (firm lumps under the skin) can develop.
-
Pathogenesis: The autoimmune attack in RA is primarily directed against the synovial membrane, which lines the joints. Autoantibodies, particularly rheumatoid factor (RF) and anti-citrullinated protein antibodies (ACPA), bind to citrullinated proteins in the joints, forming immune complexes that trigger inflammation. This leads to the activation of T-cells and the release of pro-inflammatory cytokines, such as TNF-alpha, which further amplify the immune response and cause damage to the cartilage and bone.
-
Diagnosis: Diagnosis is based on clinical symptoms, laboratory tests for rheumatoid factor and anti-CCP antibodies, and imaging studies showing joint erosion or inflammation.
-
Treatment: Early diagnosis and treatment are crucial to preventing long-term joint damage. Treatment options for RA include disease-modifying antirheumatic drugs (DMARDs) like methotrexate, which help reduce disease progression. Biologic agents that target specific immune molecules, such as TNF inhibitors, are also commonly used to control inflammation.
5. Conclusion
Autoimmunity occurs when the body’s immune system mistakenly targets its own tissues, leading to a range of diseases that can affect different organs and systems. Both systemic lupus erythematosus (SLE) and rheumatoid arthritis (RA) are examples of autoimmune disorders where immune cells and autoantibodies attack healthy tissues, causing inflammation, pain, and damage. While the exact causes of autoimmunity remain unclear, these conditions are generally managed with medications aimed at suppressing the immune response and alleviating symptoms. Although there is no cure for autoimmune diseases, early diagnosis and appropriate treatment can significantly improve outcomes for individuals affected by these chronic conditions.
b) Describe the steps involved in the immunoelectrophoresis technique.
Answer:
1. Introduction to Immunoelectrophoresis
Immunoelectrophoresis is a laboratory technique used to separate and identify proteins, specifically immunoglobulins or antibodies, based on their electrophoretic mobility and their ability to react with specific antibodies. It combines the principles of electrophoresis, a method for separating molecules based on size, charge, and other properties, with immunodiffusion, which involves the diffusion of antibodies and antigens to form precipitates. Immunoelectrophoresis is primarily used in clinical laboratories for diagnosing diseases such as multiple myeloma, nephrotic syndrome, and autoimmune disorders. It can help detect abnormal protein levels and identify specific proteins involved in disease processes.
2. Principle of Immunoelectrophoresis
The technique is based on the principle that when a sample containing proteins is subjected to an electric field in a gel, different proteins will migrate at different rates depending on their charge and size. Once the proteins have been separated by electrophoresis, a second step is performed in which antibodies (or antisera) are introduced into the gel, allowing them to react with their specific antigens (proteins), forming a precipitation line. This helps to identify the presence of specific proteins or immunoglobulins by the formation of a visible precipitate.
Immunoelectrophoresis is often used in the analysis of serum proteins for the diagnosis of various conditions such as autoimmune diseases, paraproteinemias, and protein abnormalities.
3. Preparation of the Gel
The first step in the immunoelectrophoresis technique is to prepare the agarose or agar gel. The gel is typically prepared by dissolving agarose or agar in a buffer solution and allowing it to set in a horizontal electrophoresis tray. The buffer is used to maintain a stable pH during the electrophoresis process. After the gel is set, a series of wells are created for loading the sample (containing proteins) and antiserum (which contains antibodies against specific proteins).
The gel must be of the appropriate concentration to allow the proteins to migrate properly. The concentration of agarose in the gel can vary depending on the size of the proteins being analyzed. Lower concentrations are typically used for larger proteins, while higher concentrations are used for smaller proteins.
4. Application of Sample and Electrophoresis
After the preparation of the gel, the next step is to load the sample into the wells. The sample, usually a serum or other biological fluid, is loaded into the well at one end of the gel. The gel is then subjected to an electric field.
- Electrophoresis: When the electric field is applied, proteins in the sample begin to migrate through the gel. The migration occurs according to the charge of the proteins; positively charged proteins will migrate towards the negative electrode (anode), while negatively charged proteins will migrate towards the positive electrode (cathode). Proteins with a neutral charge will not migrate significantly.
The rate at which the proteins migrate depends on factors such as their size, charge, and the viscosity of the gel. Smaller proteins typically move faster, while larger proteins will move more slowly.
The time of electrophoresis varies depending on the voltage applied and the size of the proteins. After a sufficient amount of time, the proteins will be separated into distinct bands based on their charge and size.
5. Immunodiffusion Step
Once the electrophoresis is completed and the proteins are separated into bands, the next step is the immunodiffusion process. In this step, antiserum (which contains antibodies against specific proteins of interest) is introduced to the gel.
-
Antiserum Application: A well or trough is created next to the protein bands, and a small amount of antisera is placed into the well. The antibodies in the antiserum will diffuse out from the well into the gel.
-
Antigen-Antibody Reaction: As the antibodies diffuse into the gel, they encounter the proteins separated by electrophoresis. If there are proteins in the sample that correspond to the antibodies in the antiserum, a precipitation line will form at the site where the antibody and antigen meet. This reaction is based on the formation of an immune complex, where the antibody binds to its specific antigen.
The precipitation lines indicate the presence of specific proteins that react with the antibodies. The location, intensity, and pattern of these lines are used to identify specific proteins or immunoglobulins in the sample. The strength of the reaction can provide insight into the concentration of the antigen.
6. Interpretation of Results
The final step involves interpreting the precipitation patterns and comparing them to known reference standards or to the normal patterns for the specific proteins being analyzed. The precipitation lines will appear as visible bands or arcs in the gel. These bands represent protein-antibody complexes, and their position correlates with the size and charge of the proteins involved.
Several patterns may emerge based on the sample composition:
-
Normal Patterns: In a healthy individual, the immunoelectrophoresis gel will show distinct bands that correspond to the different types of proteins in the serum, including albumin, alpha-1-globulins, alpha-2-globulins, beta-globulins, and gamma-globulins.
-
Abnormal Patterns: In cases of disease, such as multiple myeloma or nephrotic syndrome, the patterns may show abnormal spikes or monoclonal bands indicating an overproduction of a specific protein or immunoglobulin. The presence of monoclonal gammopathy or other abnormal patterns can be indicative of a disease.
7. Conclusion
Immunoelectrophoresis is a powerful technique that combines the principles of electrophoresis and immunodiffusion to analyze and identify specific proteins in biological samples. The steps involved, including gel preparation, protein separation, and immunodiffusion, allow for the identification of various proteins or antibodies present in a sample. By observing the patterns of precipitation lines, healthcare providers can diagnose and monitor various diseases, particularly those involving abnormalities in proteins such as immunoglobulins. Despite being less commonly used today due to advances in more modern techniques like immunofixation electrophoresis and enzyme-linked immunosorbent assay (ELISA), immunoelectrophoresis remains an important tool in clinical diagnostics and research.
Question:-9
a) Define antibodies and draw the structure of immunoglobulin.
Answer:
1. Introduction to Antibodies
Antibodies, also known as immunoglobulins (Ig), are proteins produced by the immune system in response to foreign invaders such as bacteria, viruses, and other pathogens. Their primary function is to identify and neutralize these foreign substances, known as antigens, by recognizing their unique structures. Antibodies are crucial components of the humoral immune response and work by binding specifically to antigens, marking them for destruction by other immune cells or preventing them from causing harm.
Each antibody is highly specific to a particular antigen, and its production is essential for adaptive immunity, allowing the body to recognize and remember pathogens. When the body encounters an antigen, the immune system produces antibodies that can target the pathogen for neutralization or destruction. These antibodies are key to providing long-term immunity, and their presence in the blood is often used as a diagnostic tool for various infections.
2. Structure of Antibodies
The structure of an antibody is highly specialized to allow it to bind specifically to antigens. Antibodies consist of four polypeptide chains: two heavy chains (H chains) and two light chains (L chains). These chains are connected by disulfide bonds and form a Y-shaped structure. Each antibody has two main regions:
-
Variable Region: The top portion of the Y-shaped antibody consists of the variable regions of both the heavy and light chains. This is the part of the antibody that binds to the antigen. The variability in this region allows antibodies to recognize a wide variety of antigens.
-
Constant Region: The bottom portion of the antibody, known as the constant region, does not vary between different antibodies of the same class. This region is involved in interactions with other components of the immune system, such as phagocytes and complement proteins, to trigger immune responses like phagocytosis or the activation of the complement cascade.
The structure of the antibody is designed to ensure that it can bind specifically to an antigen and trigger the appropriate immune response, making it an essential component of the adaptive immune system.
3. Types of Immunoglobulins
There are five main classes of immunoglobulins (Ig), each with unique functions and structures. These classes are distinguished by differences in their constant regions. The five classes of immunoglobulins are:
-
IgG: The most common antibody in the blood, IgG is involved in the secondary immune response and provides long-term immunity after infection or vaccination. It is the only antibody class that can cross the placenta to provide passive immunity to the fetus.
-
IgA: Found primarily in mucosal areas, such as the respiratory and gastrointestinal tracts, as well as in secretions like saliva, tears, and breast milk, IgA plays a key role in protecting mucosal surfaces from pathogens.
-
IgM: The first antibody produced during an immune response, IgM is highly effective in forming immune complexes and activating the complement system. It is usually the first antibody produced upon initial exposure to an antigen.
-
IgE: Associated with allergic reactions, IgE binds to allergens and triggers the release of histamine from mast cells and basophils. It plays a central role in the immune response to parasitic infections.
-
IgD: Found on the surface of B cells, IgD is involved in the activation and regulation of B cells. Its exact role is less understood but is thought to be important in initiating immune responses.
Each immunoglobulin class has its own functional properties, making it suited to different aspects of the immune response.
4. The Y-Shaped Structure of Immunoglobulin
The Y-shaped structure of immunoglobulins is highly specialized for their role in immune defense. The antibody consists of two main functional parts:
-
Fab Region (Fragment antigen-binding): The Fab region is the arms of the Y-shaped antibody and is composed of the variable regions of both the heavy and light chains. This is the site where the antibody binds to the antigen. The unique sequence of amino acids in the variable regions of the light and heavy chains allows for the recognition of a specific antigen.
-
Fc Region (Fragment crystallizable): The Fc region is the stem of the Y-shaped structure and consists of the constant regions of the heavy chains. This region does not bind to antigens but interacts with various components of the immune system. For example, it can bind to receptors on phagocytes, triggering the process of phagocytosis, or activate the complement system to enhance the immune response.
5. Function of Antibodies
Antibodies serve several important functions in the immune system:
-
Neutralization: By binding to pathogens or their toxins, antibodies can neutralize their ability to cause harm. For example, antibodies can bind to a virus and prevent it from entering host cells.
-
Opsonization: Antibodies mark pathogens for destruction by phagocytes through a process called opsonization. The Fc region of the antibody binds to receptors on phagocytes, facilitating their engulfment and destruction of the pathogen.
-
Activation of the Complement System: The binding of antibodies to pathogens can trigger the complement system, a series of proteins that help destroy pathogens by creating pores in their membranes or by enhancing phagocytosis.
-
Agglutination and Precipitation: Antibodies can cross-link pathogens or antigens, causing them to clump together (agglutination) or form insoluble complexes (precipitation), which can then be cleared by the immune system.
These functions make antibodies a crucial part of the immune response, helping the body defend against infections and diseases.
6. Structure of Immunoglobulin: Diagram
The structure of immunoglobulin (Ig) is often depicted as a Y-shaped molecule. Below is a simplified description of its components:
-
Heavy Chain: Each immunoglobulin molecule has two heavy chains (H). These chains are long and contain both variable and constant regions.
-
Light Chain: Each immunoglobulin molecule has two light chains (L), which are shorter than the heavy chains and also consist of variable and constant regions.
-
Variable Region: This is the top part of the Y-shaped structure and includes the antigen-binding sites, which are formed by parts of both the heavy and light chains. The variability in these regions allows antibodies to bind specifically to a diverse range of antigens.
-
Constant Region: The stem and the lower parts of the arms of the Y-shape consist of the constant region, which interacts with immune cells and other components of the immune system.
[Note: A diagram of the immunoglobulin structure would typically be included here, showing the Y-shape with labels for the Fab regions, Fc region, heavy chains, and light chains.]
7. Conclusion
Antibodies, or immunoglobulins, are key components of the immune system that help defend the body against infections and diseases. They recognize and bind to specific antigens, neutralizing or marking them for destruction. The structure of antibodies is intricately designed for antigen binding, with the variable regions responsible for recognizing diverse antigens and the constant region interacting with other immune components. The different classes of immunoglobulins, including IgG, IgA, IgM, IgE, and IgD, each play distinct roles in the immune response, contributing to the body’s defense mechanisms in specific ways. Understanding the structure and function of antibodies is essential for comprehending how the immune system protects the body from harmful invaders.
b) Explain the significance of the different classes of antibodies.
Answer:
1. Introduction to Antibodies and Their Role in Immunity
Antibodies, also known as immunoglobulins (Ig), are specialized proteins produced by B lymphocytes (B cells) in response to foreign substances called antigens. These antigens can be parts of pathogens such as bacteria, viruses, or other harmful molecules. Once produced, antibodies play a central role in the adaptive immune system, which provides the body with specific immunity against diseases it has previously encountered.
There are five major classes of antibodies, each of which has a distinct structure and function. These classes—IgG, IgA, IgM, IgE, and IgD—are categorized based on differences in their constant regions. Understanding the significance of these different classes helps in recognizing how the immune system tailors its response to various threats.
2. Immunoglobulin G (IgG)
IgG is the most abundant class of antibody in the blood and extracellular fluid, representing approximately 75-80% of all immunoglobulins. It plays a significant role in the body’s secondary immune response and provides long-term immunity after exposure to an antigen.
-
Function: IgG antibodies are highly effective in neutralizing toxins and viruses. They are capable of crossing the placenta, providing passive immunity to the fetus, which is crucial in the early stages of life before the infant’s immune system is fully developed. IgG can also activate the complement system, enhancing the immune response by triggering inflammation and the destruction of pathogens.
-
Significance: The presence of IgG antibodies in the blood can indicate prior exposure to an infection or vaccination, making them useful in diagnostic tests for immune memory.
3. Immunoglobulin A (IgA)
IgA is the primary antibody found in mucosal areas, including the respiratory, gastrointestinal, and urogenital tracts. It is also present in saliva, tears, breast milk, and nasal secretions. IgA plays a key role in mucosal immunity, protecting the body’s epithelial surfaces from infection.
-
Function: IgA helps to neutralize pathogens, such as viruses and bacteria, by preventing their attachment to mucosal surfaces. It also plays a role in preventing the invasion of harmful microorganisms into the body’s internal tissues by blocking their adherence to epithelial cells.
-
Significance: Because IgA is secreted into mucosal areas, it is essential for protecting the body’s first line of defense against pathogens that enter through the respiratory or gastrointestinal tracts. The presence of IgA in breast milk provides newborns with passive immunity, protecting them against infections in the early months of life.
4. Immunoglobulin M (IgM)
IgM is the largest antibody and the first antibody produced during an initial exposure to an antigen. It is mainly found in the bloodstream and accounts for about 5-10% of total immunoglobulins. IgM is the first line of defense during a primary immune response.
-
Function: IgM is highly effective in forming immune complexes, which are clusters of antigens bound to antibodies. These complexes are essential for activating the complement system, which enhances the immune response and helps clear pathogens. IgM can bind to multiple antigens simultaneously, forming large complexes that can effectively neutralize pathogens.
-
Significance: The presence of IgM in the blood indicates an acute or recent infection. It is used in diagnostic tests to detect new or current infections, as it is the antibody produced during the first phase of the immune response.
5. Immunoglobulin E (IgE)
IgE is the least abundant antibody class in the blood but is critical in the immune response to allergic reactions and parasitic infections. IgE is primarily found on the surface of mast cells and basophils, two types of white blood cells involved in inflammatory responses.
-
Function: When IgE binds to specific allergens, it triggers the release of histamine and other inflammatory mediators from mast cells and basophils, leading to the characteristic symptoms of allergies, such as itching, swelling, and bronchoconstriction. IgE also plays a role in defending against parasitic infections by promoting eosinophil activation.
-
Significance: IgE-mediated immune responses are central to allergic diseases like hay fever, asthma, and anaphylaxis. Elevated levels of IgE are often seen in individuals with these conditions. In parasitic infections, IgE helps mobilize the immune system to combat helminths and other parasites.
6. Immunoglobulin D (IgD)
IgD is found in very small amounts in the blood and is primarily present on the surface of B cells, where it functions as a receptor for antigens. IgD is often considered the least understood of the five antibody classes, as its precise role in immunity is not fully elucidated.
-
Function: The primary role of IgD is to assist in the activation of B cells. When B cells encounter an antigen, the binding of IgD to the antigen helps signal the cell to initiate an immune response. IgD also plays a role in regulating the balance of immune responses and in the early stages of the immune response.
-
Significance: IgD is important in the activation and differentiation of B cells into antibody-secreting plasma cells. It is also involved in fine-tuning the immune response to ensure that the body reacts appropriately to pathogens without causing excessive inflammation.
7. Conclusion
The five classes of immunoglobulins—IgG, IgA, IgM, IgE, and IgD—play distinct but complementary roles in the immune system, contributing to the body’s defense against infections and harmful substances. IgG is crucial for long-term immunity and can neutralize pathogens and activate complement, while IgA protects mucosal surfaces and provides passive immunity to newborns. IgM is the first responder during infections, signaling acute immune activity. IgE is key to allergic responses and protection against parasites, while IgD regulates B cell activation and immune responses.
Each class of antibody has evolved to handle specific immune challenges, ensuring the body’s immune system is equipped to address a broad range of pathogens. By understanding these antibodies and their functions, scientists and healthcare professionals can develop more effective treatments and diagnostic tools for a variety of immune-related diseases.
Question:-10
How is the classical complement pathway activated? Discuss with a suitable diagram.
Answer:
1. Introduction to the Classical Complement Pathway
The classical complement pathway is one of the primary mechanisms through which the immune system fights infections and clears damaged or apoptotic cells. The complement system is a complex network of proteins that work together to defend the body against pathogens, promote inflammation, and enhance the immune response.
The classical complement pathway is triggered when antibodies (specifically IgM and IgG) bind to antigens on the surface of pathogens or damaged cells. This binding activates a cascade of reactions that ultimately lead to the destruction of the target pathogen, either by phagocytosis, membrane attack complex (MAC) formation, or inflammation. Understanding how the classical pathway is activated is essential for understanding how the immune system responds to infection and tissue damage.
2. Components of the Complement System
The complement system consists of a series of plasma proteins that are produced mainly in the liver and circulate in an inactive form. There are over 30 different complement proteins, which are divided into three main pathways: the classical, alternative, and lectin pathways. In this discussion, we focus on the classical pathway, which is the most well-studied and the first to be described.
The key components involved in the classical complement pathway include:
-
C1 Complex: The first component of the classical pathway, C1 is composed of three subunits: C1q, C1r, and C1s. C1q binds to the antibody-antigen complex, and this binding activates the C1r subunit, which in turn activates C1s.
-
C2 and C4: Once activated, C1s cleaves the C2 and C4 proteins, forming the C3 convertase, a crucial step in the complement cascade.
-
C3: The central molecule in the complement system, C3 is cleaved into C3a and C3b. C3b binds to the pathogen surface and promotes phagocytosis, while C3a promotes inflammation by acting as a potent anaphylatoxin.
-
C5, C6, C7, C8, and C9: These components come together to form the membrane attack complex (MAC), which creates pores in the pathogen’s membrane, leading to its destruction.
The classical pathway is activated when antibodies bind to antigens on the surface of pathogens or other target cells, setting off a series of steps that eventually lead to pathogen elimination.
3. Activation of the Classical Complement Pathway
The activation of the classical complement pathway involves several key steps. These steps include the recognition of the pathogen by antibodies, the binding of C1q to the antibody-antigen complex, and the subsequent activation of a series of complement proteins.
-
Step 1: Antigen Recognition and Antibody Binding: The classical complement pathway begins when an antibody, typically IgM or IgG, binds to a specific antigen on the surface of a pathogen. This binding occurs through the variable region of the antibody, which recognizes a unique structure on the antigen.
-
Step 2: Binding of C1q to the Antibody-Antigen Complex: The next step involves the C1 complex, which consists of three subunits: C1q, C1r, and C1s. C1q binds to the Fc region of the antibody that is bound to the antigen. This interaction causes a conformational change in C1q, activating the associated C1r and C1s subunits.
-
Step 3: Activation of C1r and C1s: Upon activation, C1r cleaves and activates C1s. Activated C1s then cleaves C4 into C4a and C4b. C4b binds to the pathogen surface, while C4a acts as a potent anaphylatoxin, contributing to inflammation.
-
Step 4: Formation of C3 Convertase (C4b2a): Activated C1s also cleaves C2 into C2a and C2b. C2a binds to C4b on the pathogen surface to form the C3 convertase complex (C4b2a). This enzyme complex is responsible for cleaving C3 into C3a and C3b.
-
Step 5: Cleavage of C3 and Formation of C3b: The cleavage of C3 into C3a and C3b is a critical step in the classical pathway. C3a acts as a chemotactic factor and an anaphylatoxin, promoting inflammation and recruiting immune cells like neutrophils to the site of infection. C3b binds to the pathogen surface, opsonizing it for phagocytosis and also forming the C5 convertase.
-
Step 6: Formation of C5 Convertase (C4b2a3b): The next step involves the assembly of the C5 convertase, which consists of C4b2a3b. This enzyme cleaves C5 into C5a and C5b. C5a is another anaphylatoxin that promotes inflammation, while C5b plays a key role in the formation of the membrane attack complex (MAC).
-
Step 7: Formation of the Membrane Attack Complex (MAC): The final step in the classical complement pathway is the assembly of the membrane attack complex (MAC). C5b binds to C6 and C7, forming a complex that attaches to the pathogen surface. The C5b-7 complex then binds to C8, which initiates the polymerization of C9 molecules. The C9 molecules form a pore in the pathogen’s membrane, leading to its lysis and death.
4. Diagram of the Classical Complement Pathway
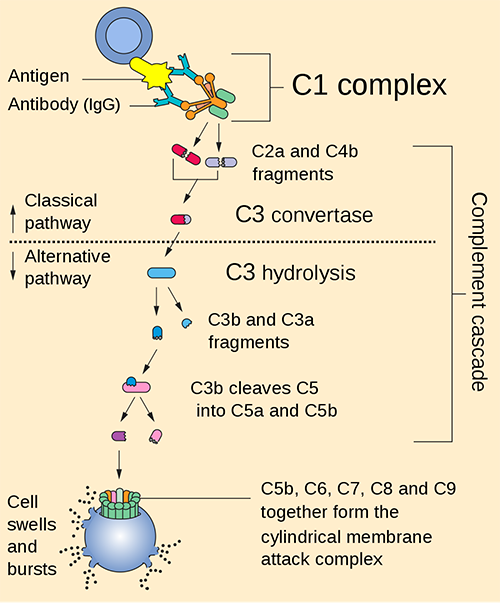
5. Conclusion
The classical complement pathway is a crucial part of the immune system’s ability to recognize and eliminate pathogens. It is triggered by the binding of antibodies (IgG or IgM) to antigens on the surface of pathogens, initiating a cascade of events that ultimately leads to the formation of the membrane attack complex (MAC) and the destruction of the pathogen. This pathway also promotes inflammation and enhances the ability of phagocytes to clear the pathogens. The sequential activation of complement proteins, from C1q to the MAC, ensures an effective and coordinated immune response. Understanding the classical complement pathway is essential for exploring its therapeutic potential in combating infections and modulating immune-related diseases.