BZYET-143 Solved Assignment
-
Which structural, developmental and behavioristic characteristics make insects highly successful organisms on Earth? Explain.
-
Write short notes on the following:
i) Mouthpart modifications in insects
ii) Types of antennae in insects
iii) Leg modification in insects
iv) Typical structure of the wing -
Discuss the reproduction and metamorphosis in insects.
-
Distinguish between the following insect orders based on the clue given in parentheses.
i) Mallophaga and Anoplura (Mouth parts)
ii) Heteroptera and Homoptera (Forewings)
iii) Diptera and Strepsiptera (Reduced wings)
iv) Isoptera and Hymenoptera (Joining of thorax and abdomen) -
a) Explain vector-pathogen relationship.
b) Differentiate between:
i) Propagative Transmission and Cyclopropagative Transmission
ii) Cyclodevelopmental Transmission and Vertical Transmission -
Name four insect orders which are of medical importance. Write at least one conspicuous feature and three examples of each order.
-
Explain the epidemiology of malarial parasite.
-
Draw a labeled diagram of:
a) Life cycle of Aedes mosquito
b) Dengue transmission Cycle
c) Zika Transmission Cycle -
Write short notes on:
a) Traps used for controlling houseflies
b) Cultural control of Musca
c) Chemical control of housefly
d) Myiasis
10. a) Explain the concept of Integrated Vector Management.
b) How can the disease vectors be genetically manipulated to reduce their population?
d) Myiasis
10. a) Explain the concept of Integrated Vector Management.
b) How can the disease vectors be genetically manipulated to reduce their population?
Answer:
Question:-1
Which structural, developmental and behavioristic characteristics make insects highly successful organisms on Earth? Explain.
Answer:
1. Introduction to Insect Success
Insects are the most diverse and abundant group of organisms on Earth, with over 1 million described species and possibly as many as 10 million total species. Their success in a wide range of environments, from the deepest caves to the highest mountains, can be attributed to a combination of structural, developmental, and behavioral adaptations. These adaptations have allowed insects to thrive for hundreds of millions of years, outliving many other species and continuing to play critical roles in ecosystems worldwide. To understand why insects are so successful, we must examine the unique characteristics that have contributed to their ecological dominance.
2. Structural Characteristics
Insects have evolved a variety of structural features that give them an advantage over other organisms, enabling them to survive, reproduce, and exploit many different ecological niches.
-
Exoskeleton: One of the most important structural features of insects is their exoskeleton—a rigid external covering made of chitin. This exoskeleton provides several benefits:
- Protection: It acts as a barrier against physical damage, predators, and dehydration, especially in harsh environments.
- Support: It supports the insect’s body and internal organs, allowing them to maintain their shape and function despite external pressures.
- Moulting: The ability to shed and regrow their exoskeleton allows insects to grow without being constrained by their body size. This process, known as ecdysis, enables them to adapt to their environment as they develop through different life stages.
-
Small Size: Insects are typically small, which confers multiple advantages:
- Energy Efficiency: Smaller body sizes require fewer resources to sustain. This energy efficiency allows them to thrive in environments where food may be scarce or patchy.
- Mobility: Their small size allows insects to navigate through narrow spaces, fly, or hide in places where larger animals cannot reach, giving them access to various habitats and food sources.
-
Wings: Many insects possess wings, which have been crucial for their survival and proliferation:
- Dispersal: Wings allow insects to travel over large distances, finding new habitats and escaping from predators. Flying also enables them to access food sources that may be out of reach for non-flying organisms.
- Reproductive Success: Flying helps insects find mates more efficiently, increasing the chances of successful reproduction.
-
Segmented Body: Insects have a segmented body structure, which contributes to their flexibility and specialization. The three primary body regions—the head, thorax, and abdomen—are distinct and each serves specialized functions.
- Head: Contains sensory organs such as compound eyes, antennae, and mouthparts adapted for feeding on specific food sources.
- Thorax: Houses the legs and wings, essential for movement and dispersal.
- Abdomen: Contains vital organs for digestion, reproduction, and respiration.
3. Developmental Characteristics
Insects undergo a variety of developmental processes that contribute to their success in different environments. Their life cycles, in particular, are marked by unique developmental stages that provide significant advantages.
-
Metamorphosis: Most insects undergo some form of metamorphosis, a process where they develop through distinct stages from egg to adult. There are two types of metamorphosis:
- Complete Metamorphosis: Insects like butterflies, beetles, and flies undergo four stages: egg, larva, pupa, and adult. This life cycle allows for specialization at different stages of life. For example, the larva may feed on a particular resource, while the adult may feed on something else or focus on reproduction.
- Incomplete Metamorphosis: Insects like grasshoppers and cockroaches go through three stages: egg, nymph, and adult. The nymph resembles the adult but lacks wings and reproductive structures. This type of metamorphosis allows for a quicker transition to adulthood, especially in environments where resources are abundant.
-
Reproductive Strategy: Insects have developed diverse reproductive strategies that enable them to maximize their offspring survival:
- High Reproductive Rate: Many insects produce large numbers of offspring in a short period, which increases the likelihood of species survival. For example, a single female mosquito can lay hundreds of eggs in a single cycle.
- Parental Investment: Some insects, like certain species of beetles and cockroaches, provide care for their young. This behavior ensures that a higher percentage of offspring survive to adulthood.
- Asexual Reproduction: Some insect species can reproduce asexually through parthenogenesis, a process where females produce offspring without mating. This allows populations to grow rapidly when mates are scarce.
4. Behavioral Characteristics
The behavior of insects is another crucial factor in their success. Behavioral adaptations allow insects to exploit a wide range of resources, protect themselves from predators, and reproduce effectively.
-
Social Organization: Many insects, particularly hymenopterans (bees, ants, wasps), exhibit complex social structures:
- Division of Labor: In social insects, individuals perform different tasks based on their roles within the colony. Worker ants may forage for food, while queen ants focus on reproduction, and soldier ants protect the colony. This division of labor enhances the efficiency of the colony.
- Cooperation: Social insects work together to gather food, build nests, and care for offspring. In bees, cooperation is essential for the production of honey and the pollination of plants, while ants can collectively overcome larger prey or defend their nests against intruders.
- Communication: Insects such as bees, ants, and termites use various methods of communication, such as pheromones, sound, and tactile signals, to coordinate their activities and share information about food sources, danger, and reproduction.
-
Foraging and Feeding Behavior: Insects have highly specialized feeding habits:
- Pollination: Many insects, like bees and butterflies, play a key role in the pollination of flowering plants. This mutualistic relationship benefits both the insects (which feed on nectar) and the plants (which reproduce via cross-pollination).
- Predation and Herbivory: Insects also act as predators or herbivores, contributing to the regulation of other species. Ladybugs, for example, feed on aphids, controlling aphid populations. Other insects, like caterpillars, consume plants, affecting plant populations and ecosystem dynamics.
-
Defense Mechanisms: Insects have evolved various defensive behaviors and physical adaptations to protect themselves from predators:
- Camouflage: Some insects, like stick insects and moths, have evolved camouflage to blend into their environment and avoid detection.
- Chemical Defense: Many insects, such as beetles and ants, secrete toxic chemicals or use venom to defend themselves from predators.
- Flight: Insects like dragonflies and moths use flight as a means of escaping predators or finding new habitats.
5. Ecological and Evolutionary Factors
Insects also benefit from their role in ecosystems and their ability to adapt to changing conditions, making them highly resilient organisms.
-
Niche Specialization: Insects occupy a wide range of ecological niches, from pollinators and decomposers to predators and herbivores. This diversity in roles allows insects to exploit nearly every available resource on Earth, reducing competition and promoting species diversification.
-
Adaptive Radiation: Insects have undergone significant evolutionary diversification, allowing them to adapt to various habitats. For example, beetles are one of the most diverse insect families, having adapted to nearly every type of environment and ecological role.
6. Conclusion
The remarkable success of insects can be attributed to their combination of structural, developmental, and behavioral characteristics that have allowed them to adapt to a vast range of environments and ecological roles. Their exoskeleton provides protection and support, while their small size and wings facilitate mobility and dispersal. The developmental strategies, including metamorphosis and high reproductive rates, allow insects to adapt quickly to environmental changes. Insects’ behavioral adaptations, such as complex social structures, specialized feeding, and efficient defense mechanisms, further contribute to their evolutionary success. By filling a variety of ecological niches and evolving complex strategies for survival, insects continue to be the dominant organisms on Earth.
Question:-2
Write short notes on the following:
i) Mouthpart modifications in insects
Answer:
i) Mouthpart Modifications in Insects
Insects have a wide variety of mouthpart modifications that allow them to feed on different food sources, from plants to animals. These adaptations are crucial for their survival and have evolved to cater to the specific needs of each species. The basic mouthparts of insects consist of labrum, mandibles, maxillae, labium, and hypopharynx. Based on the feeding habits, the mouthparts can be modified into different forms:
-
Chewing Mouthparts: Found in insects like grasshoppers and beetles, these are adapted for biting and chewing solid food. The mandibles are well-developed and used to cut or grind food.
-
Piercing and Sucking Mouthparts: Seen in insects like mosquitoes and lice, these mouthparts are modified into long, needle-like structures that pierce the skin or plant tissues to feed on fluids such as blood or sap. The mandibles are modified into stylets.
-
Siphoning Mouthparts: Found in butterflies and moths, these mouthparts are modified into a long, coiled tube (proboscis) used for sucking nectar from flowers.
-
Sponging Mouthparts: Insects like houseflies and blowflies have sponging mouthparts that allow them to soak up liquid food by creating a spongy surface for absorption.
-
Lapping Mouthparts: These are found in bees and are specialized for lapping up liquids, such as nectar. The labium forms a long, flexible tongue used to lap liquids.
These modifications enable insects to efficiently exploit various food resources, making them highly adaptable in different environments.
ii) Types of antennae in insects
Answer:
ii) Types of Antennae in Insects
Insects possess antennae that serve as sensory organs to detect environmental stimuli like chemicals, vibrations, and wind. Depending on the insect’s environment and needs, the antennae are adapted in different shapes and structures. There are several types of antennae in insects:
-
Filiform Antennae: These are thread-like antennae found in beetles and cockroaches. They are long and slender, with segments of equal length. Filiform antennae are highly sensitive to touch and vibration.
-
Moniliform Antennae: These are bead-like antennae found in termites and some beetles. The segments are globular, resembling a string of beads. They help in sensing vibrations or air currents.
-
Serrate Antennae: Characterized by jagged or saw-like edges, serrate antennae are common in beetles (like fireflies). These are used to sense environmental cues such as sound and chemicals.
-
Plumose Antennae: These are bushy or feathery antennae found in moths and male mosquitoes. They are covered in fine hairs and are highly sensitive to air currents and pheromones, aiding in mate location.
-
Geniculate Antennae: These antennae have a sharp bend, similar to a knee joint. They are found in ants and some beetles and are important for detecting pheromones and other environmental stimuli.
-
Lamellate Antennae: These antennae are flat and have leaf-like structures. They are common in beetles (like scarabs) and help in detecting vibrations or chemical signals.
iii) Leg modification in insects
Answer:
iii) Leg Modification in Insects
Insects exhibit a wide range of leg modifications that suit their lifestyles, environments, and modes of movement. The typical insect leg has five segments: coxa, trochanter, femur, tibia, and tarsus. Modifications to these segments have allowed insects to evolve specialized forms of locomotion:
-
Walking Legs: Found in most insects, these legs are adapted for walking on land or other surfaces. Insects like cockroaches and beetles have long, sturdy legs that enable efficient movement.
-
Jumping Legs: Found in grasshoppers and froghoppers, these legs are adapted for powerful jumping. The femur (thigh) is especially large and strong to store energy that is quickly released to propel the insect into the air.
-
Swimming Legs: Insects like water beetles and water bugs have legs modified for swimming. These legs are often flattened and fringed with hairs, aiding in propulsion through water.
-
Grasping Legs: Seen in mantids, these legs have spines and are modified for capturing prey. The femur and tibia are often spiny or hooked, allowing the insect to seize and hold its prey.
-
Digging Legs: Insects like mole crickets have forelegs modified for digging. These legs are wide, flattened, and strong to enable burrowing into soil or other substrates.
-
Pollen-Collecting Legs: Found in bees, these legs are modified with special hairs or structures that help collect pollen from flowers for food storage or offspring.
iv) Typical structure of the wing
Answer:
iv) Typical Structure of the Wing
Insects are the only animals that possess true flight, and their wings are essential for their mobility and success. The typical structure of an insect wing is adapted to support flight, but different insect species have specialized wing structures depending on their environment and lifestyle.
-
Basic Structure: Insects typically have two pairs of wings, the forewings and hindwings, although some insects (like flies) only have one pair. The wings are made of a thin, flexible membrane supported by a network of veins. These veins are composed of chitin and provide structural integrity to the wing.
-
Wing Veins: The veins in insect wings are responsible for their rigidity and help in blood circulation (hemolymph) to supply the wings with nutrients. The veins create a complex pattern, which varies between species. The major veins in a wing include:
- Costa (leading edge of the wing)
- Subcosta
- Radius
- Media
- Cubitus
- Anal veins
-
Flight Muscles: The wings are powered by specialized flight muscles that attach to the thorax. In some insects, like flies, the flight muscles are directly responsible for wing movement, while in others like beetles, the muscles work to control the wing movements indirectly via the thoracic exoskeleton.
-
Modified Wings: In some species, wings are modified for functions other than flight:
- Halteres: In flies, the hindwings are reduced to small, club-shaped structures known as halteres, which serve as stabilizers during flight.
- Elytra: In beetles, the forewings are modified into hard, protective coverings (elytra) that protect the hindwings, which are used for flight.
-
Wing Venation and Flexibility: The pattern and flexibility of wing veins allow for precise flight control. In dragonflies, wings are highly flexible, allowing for intricate maneuvers, while in butterflies, wings are more rigid for gliding flight.
In conclusion, insect wings are marvels of evolutionary design, offering a combination of structural complexity, flexibility, and strength to support the demands of flight.
Question:-3
Discuss the reproduction and metamorphosis in insects.
Answer:
1. Introduction to Reproduction and Metamorphosis in Insects
Insects exhibit remarkable diversity in their reproductive strategies and developmental processes, making them one of the most successful groups of organisms on Earth. Their ability to adapt to different environmental conditions, coupled with their unique methods of reproduction and metamorphosis, has enabled insects to thrive in virtually every habitat. The reproductive strategies and metamorphic processes vary widely across insect species, but they all serve the purpose of ensuring the continuation of the species. In this comprehensive explanation, we will discuss the various mechanisms of insect reproduction and the different forms of metamorphosis that insects undergo during their lifecycle.
2. Reproductive Strategies in Insects
Insect reproduction is highly diverse, ranging from simple asexual reproduction to complex sexual behaviors involving intricate mating rituals. The reproductive processes of insects are generally adapted to the species’ ecological needs, ensuring the survival and dissemination of their genes.
-
Sexual Reproduction: Most insects reproduce sexually, involving the union of male and female gametes (sperm and eggs). In many species, the male produces sperm, which is transferred to the female through specialized reproductive structures such as the genitalia. The female then stores the sperm in specialized organs called spermathecae and uses it to fertilize her eggs as she lays them.
- Copulation: Mating in insects can vary greatly. For example, in beetles and cockroaches, copulation is a brief process, while in mantids, the male often performs a complex courtship ritual before mating, which may be followed by sexual cannibalism.
- Mating Systems: Insects exhibit diverse mating systems such as monogamy, polygyny (one male mates with multiple females), and polyandry (females mate with multiple males). The mating system varies based on environmental conditions, the availability of mates, and evolutionary pressures.
- Parthenogenesis: Some insect species, such as aphids and certain bees, are capable of parthenogenesis, a form of asexual reproduction where females produce offspring without the need for fertilization by a male. Parthenogenesis allows rapid population growth, especially in favorable environments.
-
Egg Laying and Parental Care: After mating, females typically lay their eggs in protected environments to enhance the chances of offspring survival. In some species, females show parental care by guarding their eggs, like the stick insects or cockroaches that protect their young until they hatch.
3. Mechanism of Metamorphosis in Insects
Metamorphosis is the process by which insects undergo dramatic transformations in their development, from juvenile to adult stages. Insects can exhibit two primary types of metamorphosis: complete metamorphosis and incomplete metamorphosis. These processes ensure that insects can efficiently adapt to their environment at various life stages, often allowing the larvae to exploit different ecological niches than the adults.
-
Complete Metamorphosis (Holometabolism): Insects that undergo complete metamorphosis pass through four distinct life stages: egg, larva, pupa, and adult. This type of metamorphosis is found in approximately 85% of all insect species and is particularly advantageous in environments where food sources are seasonal or varied.
- Egg: The life cycle begins with the egg, which is typically laid by the female in a suitable environment for the developing larvae.
- Larva: After hatching, the insect enters the larval stage. Larvae are usually worm-like and focused primarily on feeding and growth. The larval stage is marked by high metabolic activity and the accumulation of resources needed for the next stage of development. Examples include the caterpillar in moths and butterflies or the grub in beetles.
- Pupa: Once the larva has grown sufficiently, it enters the pupal stage, where it undergoes a significant transformation, or metamorphosis, within a protective cocoon or chrysalis. During this stage, the insect reorganizes its internal and external structures. In moths, for example, the caterpillar transforms into a butterfly inside the pupa.
- Adult: The adult emerges from the pupa as a fully formed insect capable of reproduction. Adults are typically more mobile than larvae and often specialize in different ecological roles. For instance, a caterpillar feeds on leaves, while the butterfly (adult) focuses on reproduction and pollination.
-
Incomplete Metamorphosis (Hemimetabolism): Insects that undergo incomplete metamorphosis pass through three primary life stages: egg, nymph, and adult. This process is seen in insects like grasshoppers, cockroaches, and dragonflies.
- Egg: Similar to complete metamorphosis, the insect begins life as an egg, which hatches into a nymph.
- Nymph: The nymph resembles a small adult but lacks wings and reproductive organs. The nymph continues to grow through successive molts, shedding its exoskeleton each time to allow for growth. Unlike complete metamorphosis, the nymph undergoes gradual development, and each molt brings it closer to adult form.
- Adult: Once the nymph has grown enough, it molts one final time to become an adult, capable of reproduction. In insects like grasshoppers, the adult stage includes wings and fully developed reproductive organs.
-
Ametabolous Development: Some insects, such as silverfish, exhibit ametabolous development, where there is little to no change between immature and adult stages. These insects grow by simply increasing in size with each molt, without any drastic transformation like metamorphosis.
4. Factors Influencing Metamorphosis
Metamorphosis is influenced by several factors, including genetics, environmental conditions, and hormonal regulation. These factors determine when and how insects transition from one life stage to the next.
-
Environmental Factors: Temperature, humidity, and food availability can impact the timing of metamorphosis. For instance, in some species, if the larvae do not have enough food or experience adverse environmental conditions, they may delay pupation. Conversely, abundant resources may cause earlier development.
-
Hormonal Regulation: Insects rely on a set of hormones to regulate the transition between life stages. The two most important hormones involved in metamorphosis are ecdysone, which promotes molting and the shedding of the exoskeleton, and juvenile hormone, which determines the nymph’s characteristics and prevents it from reaching adult form prematurely. The balance between these hormones ensures proper development from larva to pupa to adult.
-
Genetics and Developmental Pathways: The genetic makeup of an insect plays a key role in determining its metamorphic pathway. Some species may have evolved specific genetic pathways that enable them to respond to environmental signals, ensuring that the timing of metamorphosis is optimal for survival.
5. Significance of Metamorphosis in Insect Life Cycles
Metamorphosis provides several advantages to insect species, contributing to their evolutionary success.
-
Niche Specialization: Complete metamorphosis allows larvae and adults to exploit different ecological niches. For example, a caterpillar (larva) may feed on leaves, while the butterfly (adult) may engage in pollination. This reduces competition between life stages, allowing both to thrive independently.
-
Increased Survival Rates: By having distinct life stages, insects can avoid predators that specialize in preying on one particular stage. For example, the pupal stage is often hidden and protected, reducing the chances of predation.
-
Efficient Resource Utilization: Different stages of metamorphosis often use different resources. Larvae may focus on feeding and growing, while adults may focus on reproduction. This ensures that resources are effectively used and that each stage contributes to the success of the species.
-
Adaptability: The flexibility of metamorphosis allows insects to adapt to changing environments. For example, some species can adjust the timing of their metamorphosis based on environmental factors like temperature or food availability.
6. Conclusion
Insects exhibit an extraordinary variety of reproductive strategies and metamorphic processes, which have been central to their evolutionary success. Their ability to reproduce in different ways—through sexual reproduction, parthenogenesis, or complex mating behaviors—ensures the continuation of the species. Metamorphosis, whether complete or incomplete, allows insects to diversify and specialize in their ecological roles, reducing competition and maximizing resource use. The ability of insects to adapt to their environment and thrive in diverse habitats has made them one of the most successful groups of organisms on Earth.
Question:-4
Distinguish between the following insect orders based on the clue given in parentheses:
i) Mallophaga and Anoplura (Mouth parts)
Answer:
i) Mallophaga and Anoplura (Mouth Parts)
-
Mallophaga (Chewing Mouthparts): The members of this order, commonly known as chewing lice, have mouthparts adapted for chewing. These insects feed on the feathers, skin, or hair of birds and mammals. Their mandibles are well-developed and used for biting and grinding food. Mallophaga are typically ectoparasites that infest birds and some mammals.
-
Anoplura (Sucking Mouthparts): The sucking lice in this order have highly specialized mouthparts designed for piercing and sucking blood from their hosts. Their mouthparts consist of a tube-like structure, formed by modified mandibles and maxillae, used to penetrate the skin of their host and feed on blood. Anoplura are exclusively blood-feeding ectoparasites, often found on mammals, including humans.
ii) Heteroptera and Homoptera (Forewings)
Answer:
ii) Heteroptera and Homoptera (Forewings)
-
Heteroptera (Hemelytra – Partially Hardened Forewings): In the Heteroptera group (often referred to as true bugs), the forewings are hemelytra, meaning they are partially hardened at the base and membranous at the tip. This gives them a characteristic appearance. The forewings are thick and leathery near the body, transitioning into a membranous section further out. Examples include bedbugs, stink bugs, and water bugs.
-
Homoptera (Fully Membranous Forewings): The insects in the Homoptera group, now often classified under the suborder Auchenorrhyncha or Sternorrhyncha, have fully membranous forewings. These wings are transparent and not hardened at the base. Insects like aphids, cicadas, and leafhoppers belong to this order. Homopterans are typically plant feeders, using their piercing-sucking mouthparts to extract sap.
iii) Diptera and Strepsiptera (Reduced wings)
Answer:
iii) Diptera and Strepsiptera (Reduced Wings)
-
Diptera (Halteres – Reduced Hindwings): Diptera, or true flies, have a characteristic feature: they possess halteres—modified hindwings that are small, knob-like structures used for balance during flight. The forewings are fully developed and used for flight, while the halteres act as gyroscopic organs, stabilizing the insect’s flight path. Examples include houseflies, mosquitoes, and fruit flies.
-
Strepsiptera (Reduced Forewings): In Strepsiptera, commonly known as twisted-wing parasites, the forewings are highly reduced and are modified into small, club-shaped structures. These are non-functional for flight. The hindwings, however, are large and membranous, and they are used for flight. Strepsiptera are endoparasites, often parasitizing other insects like bees and wasps.
iv) Isoptera and Hymenoptera (Joining of thorax and abdomen)
Answer:
iv) Isoptera and Hymenoptera (Joining of Thorax and Abdomen)
-
Isoptera (Loose Joining between Thorax and Abdomen): Isoptera, or termites, have a relatively loose and flexible connection between the thorax and abdomen. This allows some flexibility in their body movements, although the segmentation is generally clear. Termites are eusocial insects that live in colonies, and their anatomy is adapted for their role in wood digestion and colony organization.
-
Hymenoptera (Tight Joining between Thorax and Abdomen): Hymenoptera, including bees, wasps, and ants, have a tight and narrow connection between the thorax and abdomen, which is often referred to as a "petiole." This constriction allows the abdomen to move more freely, and the rigid connection is important for various functions, such as stinging (in some species) and efficient movement within the colony.
Question:-5
a) Explain vector-pathogen relationship.
Answer:
Introduction to Vector-Pathogen Relationship
The vector-pathogen relationship refers to the interaction between a disease-causing organism (the pathogen) and an organism that transmits the pathogen from one host to another (the vector). This relationship is fundamental to understanding how certain diseases spread and persist in populations. Vectors are typically arthropods, such as mosquitoes, ticks, and flies, which can carry pathogens between animals and humans. The study of these interactions is crucial in epidemiology, disease control, and public health strategies.
1. Vectors: Definition and Types
A vector is any living organism that can carry and transmit an infectious pathogen to another living organism. Vectors do not necessarily get sick from the pathogens they carry, but they play a critical role in spreading the disease to other organisms. Vectors are generally classified into two main categories:
-
Biological Vectors: These vectors are involved in the life cycle of the pathogen. The pathogen undergoes part of its development or reproduction in the vector before being transmitted to the next host. Examples include:
- Mosquitoes (e.g., Anopheles species for malaria, Aedes species for dengue)
- Ticks (e.g., for Lyme disease)
- Sandflies (e.g., for Leishmaniasis)
-
Mechanical Vectors: These vectors carry pathogens on their body parts but do not support the pathogen’s life cycle. They simply transport the pathogen from one host to another. Examples include:
- Flies that carry bacteria from decaying matter to food
- Cockroaches that spread bacteria like Salmonella
2. Pathogens: Types and Characteristics
Pathogens are microorganisms or agents that cause disease in their host. The key types of pathogens transmitted by vectors include:
-
Bacteria: These are single-celled organisms that can cause a range of diseases, including cholera, plague, and tuberculosis. Some bacteria can be transmitted by vectors like fleas (plague) or mosquitoes (bacteria in the genus Wolbachia).
-
Viruses: Viruses are non-living particles that can only replicate inside a host cell. Examples of vector-borne viral diseases include malaria (caused by the Plasmodium parasite, transmitted by mosquitoes), dengue fever, and Zika virus (both transmitted by Aedes mosquitoes).
-
Parasites: Many parasitic infections are transmitted through vectors, such as the protozoan parasite responsible for malaria (Plasmodium species), and Leishmania (transmitted by sandflies).
-
Fungi: Some fungal pathogens can also be transmitted by vectors, although this is less common. These typically cause plant diseases, but some, such as histoplasmosis, affect humans and animals as well.
3. Vector-Pathogen Interaction: Biological Basis
The relationship between the vector and the pathogen is dynamic and complex. The pathogen must be able to survive and, in some cases, reproduce in the vector before it can be transmitted to a new host. This interaction typically involves several steps:
-
Acquisition: The vector acquires the pathogen when it feeds on the blood or other body fluids of an infected host. For example, a mosquito picks up the Plasmodium parasite while feeding on an infected human.
-
Survival and Development in the Vector: After the pathogen enters the vector’s body, it may either survive as a dormant organism or undergo developmental stages. For example, the malaria parasite Plasmodium undergoes a complex life cycle in the mosquito, which includes stages of growth and reproduction.
-
Transmission: When the vector feeds again, it injects the pathogen into the new host through its saliva or other body fluids. For instance, a mosquito injects Plasmodium parasites into the bloodstream of a human when it feeds, transmitting malaria.
-
Incubation Period: There may be an incubation period in the vector during which the pathogen multiplies or develops before it is transmissible to a new host. This period can vary in length depending on the type of pathogen and environmental conditions.
4. Factors Influencing Vector-Pathogen Relationship
Several factors influence the efficiency and intensity of the vector-pathogen relationship:
-
Vector Competence: This refers to the vector’s ability to harbor, support, and transmit a pathogen. Factors such as the vector’s immune system, its physiology, and the environmental conditions it inhabits can affect vector competence. Not all vectors are equally effective at transmitting diseases.
-
Environmental Conditions: Climate and environmental conditions play a significant role in the survival and proliferation of both the vector and the pathogen. Warm, humid environments, for instance, may increase the breeding rate of mosquitoes and the development speed of the malaria parasite. Seasonal changes can also influence vector populations and disease outbreaks.
-
Host Availability: The presence of susceptible hosts is crucial for the transmission of the pathogen. If there are few suitable hosts for the vector to feed on, the pathogen cannot be transmitted effectively. Some vectors are opportunistic feeders and will target multiple host species.
-
Pathogen Load: The number of pathogens present in the vector can affect the probability of transmission. A vector carrying a high pathogen load is more likely to infect a new host when it feeds.
5. Examples of Vector-Pathogen Relationships
-
Malaria: The Plasmodium parasite, responsible for malaria, is transmitted by the Anopheles mosquito. The mosquito becomes infected with Plasmodium after feeding on an infected human. The parasite undergoes part of its life cycle inside the mosquito before being transmitted to another human host during subsequent mosquito bites.
-
Dengue Fever: The Aedes mosquito is the primary vector for dengue virus. The virus is picked up by the mosquito during a blood meal from an infected human and is then transmitted to another human when the mosquito feeds again.
-
Lyme Disease: The bacterium Borrelia burgdorferi, which causes Lyme disease, is transmitted by the Ixodes tick. The tick becomes infected by feeding on small mammals like rodents. After a period of incubation, the tick can transmit the bacteria to humans and other animals when it feeds.
-
Zika Virus: Similar to dengue, the Aedes mosquito also transmits the Zika virus. Zika outbreaks are more common in tropical and subtropical regions, where the vector is most prevalent.
6. Significance of the Vector-Pathogen Relationship in Disease Control
Understanding the vector-pathogen relationship is critical for the control and prevention of vector-borne diseases. Key strategies include:
-
Vector Control: Reducing the population of the vector or preventing its contact with humans is one of the primary methods for controlling vector-borne diseases. This can be achieved through the use of insecticides, biological control agents, or environmental management (e.g., eliminating mosquito breeding sites).
-
Vaccine Development: Vaccines targeting either the vector or the pathogen can prevent the disease. For example, vaccines against Plasmodium (malaria) or dengue virus aim to reduce infection rates and prevent the spread of these diseases.
-
Public Health Interventions: Educating the public on how to reduce exposure to vectors (e.g., using bed nets for mosquito protection, wearing protective clothing, or using repellents) can help reduce the transmission of vector-borne diseases.
7. Conclusion
The vector-pathogen relationship is a critical component in understanding how infectious diseases spread. Vectors, through their interactions with pathogens, facilitate the transmission of diseases to new hosts, often resulting in widespread outbreaks. Understanding these relationships is essential for devising effective strategies to control and prevent vector-borne diseases, which remain a significant global health concern.
b) Differentiate between:
i) Propagative Transmission and Cyclopropagative Transmission
Answer:
i) Propagative Transmission and Cyclopropagative Transmission
Propagative Transmission
-
Definition: In propagative transmission, the pathogen does not undergo any developmental changes within the vector. The pathogen simply replicates or increases in number within the vector without altering its form or life cycle.
-
Mechanism: The vector becomes infected by feeding on an infected host and then transmits the pathogen to a new host through subsequent feedings. The pathogen remains the same, only increasing in quantity.
-
Example:
- Malaria (Plasmodium): In some cases of malaria transmission, the Plasmodium parasite replicates within the mosquito without undergoing any significant changes in form, making the transmission a form of propagative transmission.
-
Key Features:
- Pathogen replicates within the vector.
- No developmental or morphological change of the pathogen within the vector.
- Transmission involves an increase in the number of pathogens without modification.
Cyclopropagative Transmission
-
Definition: In cyclopropagative transmission, the pathogen not only replicates but also undergoes developmental stages in the vector, leading to a change in form or maturation before it can be transmitted to a new host.
-
Mechanism: The pathogen undergoes part of its development within the vector, which may involve changes in morphology or life cycle before it can infect another host. The transmission is more complex compared to propagative transmission.
-
Example:
- Plague (Yersinia pestis): The bacterium responsible for plague undergoes developmental changes within the flea vector, including multiplication and adaptation to the flea’s digestive tract, before it can be transmitted to a new host through the flea’s bite.
-
Key Features:
- Pathogen undergoes development and multiplication within the vector.
- The pathogen changes in form or life cycle before it can infect the next host.
- Transmission involves both replication and maturation of the pathogen.
ii) Cyclodevelopmental Transmission and Vertical Transmission
Answer:
ii) Cyclodevelopmental Transmission and Vertical Transmission
Cyclodevelopmental Transmission
-
Definition: In cyclodevelopmental transmission, the pathogen undergoes developmental changes within the vector, but the pathogen’s morphology and form change significantly, typically progressing to an infective stage.
-
Mechanism: The pathogen goes through a series of developmental stages (such as larval, nymph, or other forms) within the vector. These stages often involve morphological changes that enable the pathogen to survive and be transmitted to the next host.
-
Example:
- Filariasis (Wuchereria bancrofti): The microfilariae (larval form) of the parasite undergo development within the mosquito vector before becoming infective, enabling them to infect a human host upon the mosquito’s bite.
-
Key Features:
- Pathogen undergoes developmental stages within the vector.
- There are clear changes in the form and life cycle of the pathogen.
- The vector becomes infective only after these developmental changes.
Vertical Transmission
-
Definition: Vertical transmission refers to the transmission of the pathogen from parent to offspring, typically occurring during reproduction, either during the process of fertilization or through the maternal passage of the pathogen to the offspring.
-
Mechanism: The pathogen is passed directly from the infected parent (typically the female) to the offspring during pregnancy, egg-laying, or via placental transmission. This transmission method bypasses the need for an external vector or intermediate host.
-
Example:
- HIV in Humans: HIV can be transmitted vertically from an infected mother to her baby during childbirth or through breast milk.
- Dengue Virus in Aedes Mosquitoes: The Aedes mosquito can transmit the virus vertically to its offspring through eggs, resulting in infected larvae.
-
Key Features:
- Pathogen is passed from parent to offspring.
- Transmission occurs during reproductive stages, such as pregnancy, egg-laying, or milk transfer.
- No involvement of an external vector in the transmission process.
Question:-6
Name four insect orders which are of medical importance. Write at least one conspicuous feature and three examples of each order.
Answer:
Introduction
Insects play a pivotal role in the ecosystem, with a significant impact on human health. Some insect orders are of medical importance due to their role in transmitting diseases, causing harm directly through bites, or acting as vectors for pathogens. These insects are crucial in the study of vector-borne diseases, and understanding their characteristics is essential for disease prevention and control. This paper will discuss four insect orders of medical importance, providing key features and examples of each.
1. Order: Diptera (Flies)
Conspicuous Feature: Two wings (Halteres as modified wings for balance)
Diptera, or true flies, are one of the most medically important insect orders due to their role in transmitting diseases and causing infections. They are characterized by having two wings, with the second pair of wings modified into halteres, which serve to stabilize the insect in flight.
- Examples:
- Mosquitoes (Family: Culicidae): Mosquitoes are perhaps the most well-known members of the Diptera order due to their role in transmitting diseases such as malaria, dengue fever, Zika virus, and yellow fever. They are also vectors for parasitic worms like those responsible for filariasis.
- Tsetse flies (Genus: Glossina): These flies are the vectors for trypanosomiasis, or sleeping sickness, caused by the protozoan parasite Trypanosoma.
- Houseflies (Family: Muscidae): While houseflies are not directly involved in transmitting diseases like mosquitoes, they are important mechanical vectors, spreading bacterial infections like cholera, salmonella, and shigella by carrying pathogens on their body and in their feces.
Key Features:
- Two functional wings and halteres.
- Fast and efficient flight, which aids in spreading diseases.
- Often found in urban and rural environments, where they come into contact with humans.
2. Order: Hymenoptera (Bees, Wasps, and Ants)
Conspicuous Feature: Stingers and modified mouthparts for feeding
Hymenoptera is another highly significant order, with insects like bees, wasps, and ants being known for their medical importance. They are equipped with stingers and modified mouthparts, which they use for defense, feeding, or both. Though these insects may not directly transmit diseases, they can cause a variety of allergic reactions or other health issues due to their venomous stings.
- Examples:
- Honeybees (Genus: Apis): Honeybees are crucial for pollination but can cause allergic reactions or anaphylaxis in some individuals when they sting.
- Yellowjackets (Genus: Vespula): These wasps are known for their aggressive behavior and can sting multiple times, causing pain, swelling, and sometimes severe allergic reactions.
- Fire ants (Genus: Solenopsis): Fire ants are notorious for their painful stings, which can lead to anaphylaxis in sensitive individuals. In addition, their stings can cause secondary infections when scratched.
Key Features:
- Stingers for defense or predation.
- Social behavior, with colonies often forming large nests.
- Venomous stings that can lead to allergic reactions and anaphylaxis.
3. Order: Anoplura (Sucking Lice)
Conspicuous Feature: Sucking mouthparts
Anoplura, commonly known as sucking lice, are external parasites that feed on the blood of mammals, including humans. They are medically important because they can cause direct harm through their bites and are vectors for various diseases, including epidemic typhus and trench fever.
- Examples:
- Head lice (Pediculus humanus capitis): Head lice are a common infestation in humans, especially in children. Although they do not transmit diseases, they cause itching, discomfort, and secondary bacterial infections due to scratching.
- Body lice (Pediculus humanus corporis): Body lice are vectors for epidemic typhus, caused by the bacterium Rickettsia prowazekii, and trench fever, caused by Bartonella quintana.
- Crab lice (Pthirus pubis): Crab lice are sexually transmitted parasites, primarily infesting the pubic area. They cause itching and discomfort, though they do not transmit diseases like other lice.
Key Features:
- Sucking mouthparts that pierce the host’s skin to feed on blood.
- Small, wingless insects that live on the body of the host.
- Spread in unsanitary environments and often associated with crowded living conditions.
4. Order: Hemiptera (True Bugs)
Conspicuous Feature: Piercing-sucking mouthparts
Hemiptera, also known as true bugs, are a large and diverse order of insects with piercing-sucking mouthparts used to extract fluids from plants or animals. Some members of this order are vectors for serious diseases, including Chagas disease and African sleeping sickness. They are particularly significant in tropical and subtropical regions.
- Examples:
- Kissing bugs (Family: Reduviidae): Kissing bugs are the vectors of Chagas disease, which is caused by the parasite Trypanosoma cruzi. They bite humans around the face and transmit the parasite through feces, which is then introduced into the bloodstream via the bite wound.
- Bed bugs (Cimex lectularius): Bed bugs are blood-feeding insects that cause discomfort and itching. While they are not known to transmit diseases directly, their bites can cause secondary bacterial infections.
- Cicadas (Family: Cicadidae): Though not vectors of diseases, cicadas are of medical importance because of their potential to transmit plant pathogens, which indirectly affect human food supplies.
Key Features:
- Piercing-sucking mouthparts used for feeding.
- Some species, like the kissing bug, are vectors for parasites.
- Associated with poor housing conditions, especially in tropical regions.
Conclusion
Insects, despite their small size, have a significant impact on human health, both directly and indirectly. The four insect orders discussed—Diptera, Hymenoptera, Anoplura, and Hemiptera—play a crucial role in the transmission of various diseases, as well as causing physical discomfort and allergic reactions. Understanding the features and examples of these insect orders is important for preventing infestations, controlling vector-borne diseases, and improving public health strategies worldwide. Effective control measures, such as the use of insecticides, bed nets, and environmental management, can help reduce the transmission of diseases caused by these medically important insects.
Question:-7
Explain the epidemiology of malarial parasite.
Answer:
Introduction to Malaria and Malarial Parasite Epidemiology
Malaria is one of the most significant infectious diseases worldwide, particularly in tropical and subtropical regions. It is caused by protozoan parasites belonging to the genus Plasmodium. The disease is transmitted primarily through the bites of infected female Anopheles mosquitoes, which act as vectors. Understanding the epidemiology of malaria is essential for identifying high-risk areas, developing control strategies, and eventually achieving the goal of malaria elimination. This paper delves into the various aspects of the epidemiology of malaria, including its transmission dynamics, geographical distribution, life cycle, and factors influencing the spread of the disease.
1. The Malarial Parasites: Plasmodium Species
Malaria is caused by several species of the Plasmodium parasite, each with varying degrees of impact on human health. The four main species responsible for malaria in humans are:
Plasmodium falciparum
- Most virulent and responsible for the majority of malaria-related deaths, especially in sub-Saharan Africa.
- Causes severe forms of malaria, such as cerebral malaria, and is often associated with high mortality.
Plasmodium vivax
- Prevalent in Asia, Latin America, and parts of Africa.
- Causes relapsing malaria, as the parasite can remain dormant in the liver and reactivate after months or even years.
Plasmodium ovale
- Similar to P. vivax, it causes relapsing malaria, though it is less common.
- Found primarily in West Africa.
Plasmodium malariae
- Responsible for chronic malaria that can persist for years, often with mild symptoms.
- Primarily found in sub-Saharan Africa and South Asia.
2. Transmission of Malaria
The transmission of malaria involves two main hosts: humans and the Anopheles mosquito.
Human Role in Transmission
Humans become infected when an infected Anopheles mosquito bites them. The mosquito injects sporozoites into the bloodstream, which travel to the liver where they mature. The liver stage is followed by the release of merozoites into the bloodstream, which infect red blood cells, leading to the symptoms of malaria. In some species, such as Plasmodium vivax and Plasmodium ovale, a dormant liver stage can cause relapses.
Mosquito Role in Transmission
The Anopheles mosquito plays a critical role in transmitting the parasite between humans. After ingesting the gametocytes (sexual forms of the parasite) from an infected human, the mosquito’s digestive system processes these gametocytes, allowing the parasite to develop into sporozoites in the mosquito’s salivary glands. When the mosquito bites another human, it transmits the sporozoites, continuing the transmission cycle.
3. Geographic Distribution of Malaria
Malaria is predominantly found in tropical and subtropical regions, although some cases have been reported in temperate zones. The geographical distribution is largely influenced by factors such as climate, ecology, and the presence of suitable mosquito vectors.
Tropical and Subtropical Regions
- Sub-Saharan Africa remains the most malaria-endemic region, with nearly 90% of global malaria deaths occurring here, mostly due to Plasmodium falciparum.
- Other highly endemic areas include parts of Southeast Asia, South Asia, Latin America, and the Pacific Islands.
Risk Zones
- Endemic Areas: Regions where malaria transmission is continuous and seasonal, and people are exposed to frequent mosquito bites.
- Epidemic Areas: Regions where malaria transmission occurs irregularly, often in the form of outbreaks during particular seasons due to changes in environmental conditions like rainfall and temperature.
Factors Affecting Distribution
- Climate: Warm, humid climates provide ideal conditions for the survival and reproduction of Anopheles mosquitoes.
- Altitude: Areas at higher altitudes (e.g., the highlands of East Africa and parts of the Andes) tend to have lower malaria transmission due to cooler temperatures.
- Rainfall: Mosquitoes breed in standing water, and regions with high rainfall offer more breeding sites, leading to higher transmission rates.
4. Life Cycle of the Malaria Parasite
The life cycle of the Plasmodium parasite involves multiple stages that occur both in the human host and the Anopheles mosquito.
Human Host Stages
- Sporozoite Stage: When an infected mosquito bites a human, it injects sporozoites into the bloodstream, which travel to the liver.
- Liver Stage: The sporozoites infect liver cells, where they mature and divide into merozoites. Some species (like P. vivax and P. ovale) form hypnozoites, which can remain dormant and cause relapses.
- Blood Stage: Merozoites are released from the liver and invade red blood cells. This stage causes the characteristic symptoms of malaria, such as fever and chills.
- Gametocyte Stage: Some merozoites develop into sexual forms called gametocytes, which are taken up by mosquitoes during a blood meal, completing the life cycle.
Mosquito Stages
- Gametocyte Ingestion: When a mosquito feeds on an infected human, it ingests gametocytes.
- Gametogenesis: Inside the mosquito’s stomach, the gametocytes fuse to form zygotes, which then develop into ookinetes that penetrate the mosquito’s gut wall.
- Sporozoite Formation: The ookinetes develop into sporozoites, which migrate to the mosquito’s salivary glands. The mosquito is now capable of transmitting the parasite to a new human host.
5. Factors Influencing Malaria Transmission
Several factors contribute to the dynamics of malaria transmission, influencing how widespread the disease is in a given area.
Vector Competence
- Anopheles mosquitoes are the primary vectors of malaria, but not all species of Anopheles are equally effective at transmitting the disease.
- Species such as Anopheles gambiae, Anopheles funestus, and Anopheles stephensi are highly competent vectors, while others are less efficient at transmitting the parasite.
Human Immunity
- In endemic regions, individuals who have been exposed to the parasite over time may develop partial immunity. This is more common in areas with stable malaria transmission.
- However, people with no previous exposure (e.g., travelers or newborns) are more susceptible to severe forms of malaria.
Drug Resistance
- The emergence of resistance to antimalarial drugs, such as chloroquine and artemisinin, has been a major challenge in controlling malaria. This resistance occurs due to mutations in the parasite genome that reduce the effectiveness of treatment.
Environmental Changes
- Changes in climate, land use, and the introduction of irrigation systems can create new breeding sites for Anopheles mosquitoes, increasing malaria transmission in previously low-risk areas.
6. Malaria Prevention and Control
The epidemiology of malaria emphasizes the need for robust control measures. Several strategies are employed to reduce transmission and prevent infection.
Insecticide-treated Bed Nets (ITNs)
- ITNs are one of the most effective methods for preventing mosquito bites, particularly in endemic areas. These nets help protect individuals during the night, when Anopheles mosquitoes are most active.
Indoor Residual Spraying (IRS)
- IRS involves spraying the interior walls of homes with insecticides that kill mosquitoes when they land. This reduces the number of mosquitoes in the home environment.
Antimalarial Drugs
- Preventive treatment, including the use of chemoprophylaxis, is essential for travelers and individuals at high risk of malaria in endemic regions.
- Artemisinin-based combination therapies (ACTs) remain the first-line treatment for uncomplicated malaria caused by Plasmodium falciparum.
Vaccine Development
- The development of a malaria vaccine has been a major milestone. The RTS,S/AS01 vaccine has shown promising results, though it is not yet universally available.
Conclusion
The epidemiology of malaria is influenced by complex interactions between the parasite, its vector, and the human population. Malaria continues to be a major global health challenge, particularly in tropical and subtropical regions. By understanding the factors that drive transmission, such as environmental conditions, vector competence, and human immunity, effective control measures can be developed. Strategies like insecticide-treated nets, indoor spraying, and the development of new treatments and vaccines are essential for controlling and eventually eliminating malaria worldwide.
Question:-8
Draw a labeled diagram of:
a) Life cycle of Aedes mosquito
Answer:

b) Dengue transmission Cycle
Answer:
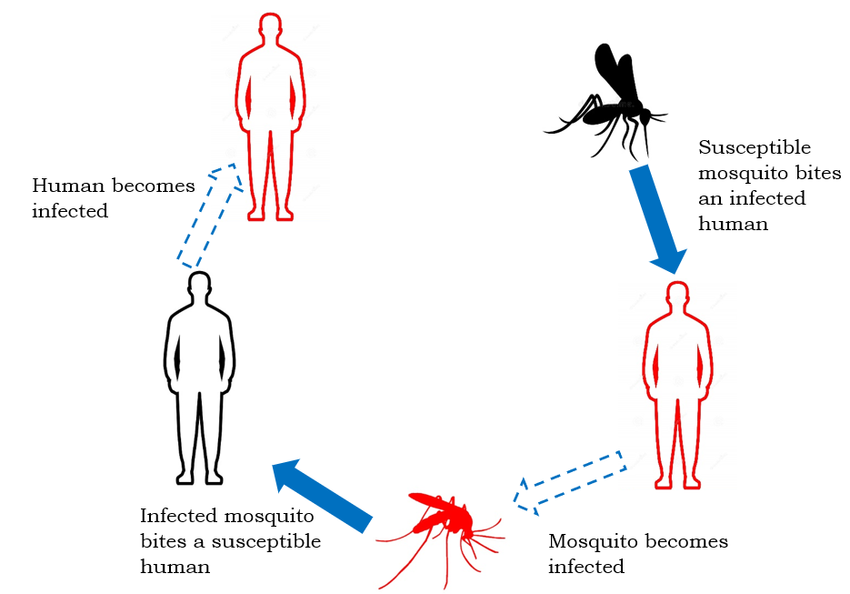
c) Zika Transmission Cycle
Answer:
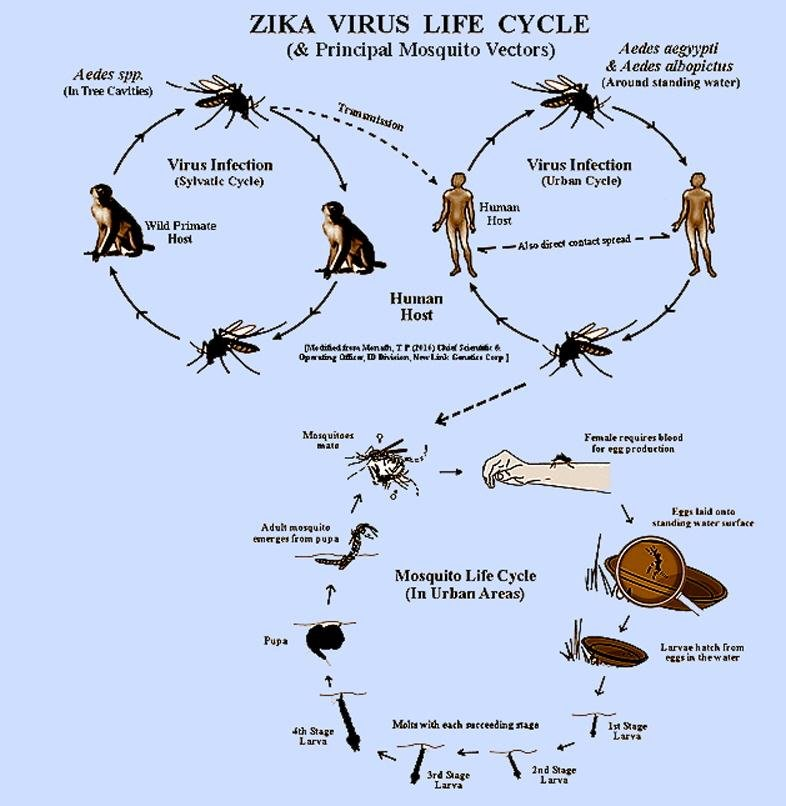
Question:-9
Write short notes on:
a) Traps used for controlling houseflies
Answer:
a) Traps Used for Controlling Houseflies
Houseflies (Musca domestica) are common pests that can spread diseases and contaminate food. Several types of traps are used for their control, particularly in urban and agricultural settings. These traps work by attracting flies through various means, such as food odors, light, or sticky surfaces. Here are some common types of traps:
-
Flypaper Traps:
Flypaper is a sticky substance coated on a strip of paper or plastic. When houseflies land on the paper, they get stuck, preventing them from flying away. These traps are effective in low-density areas but can be unsightly. -
Electric Fly Zappers:
These traps attract flies using ultraviolet (UV) light. When the flies come into contact with the light, they are zapped by an electric grid, killing them instantly. They are particularly effective for night-flying insects but can also attract beneficial insects. -
Baited Traps:
These traps use food-based attractants to lure houseflies. Some examples include homemade traps with fermenting food or commercially available bait products. The traps capture the flies once they are drawn into the container. -
Vacuum Traps:
A vacuum trap uses suction to draw flies into a container, where they are trapped. These are often used in larger, enclosed spaces like farms or food-processing areas.
Each of these traps can be used as part of an integrated pest management strategy to reduce the housefly population in specific areas.
b) Cultural control of Musca
Answer:
b) Cultural Control of Musca
Cultural control refers to agricultural or environmental practices that reduce housefly populations by eliminating or reducing the conditions that favor their breeding. Key methods of cultural control for houseflies include:
-
Sanitation:
Regular cleaning and the removal of organic waste (such as food scraps and animal manure) can significantly reduce the breeding sites for houseflies. Flies often breed in moist, decaying organic material. -
Waste Management:
Proper disposal of garbage, including sealed containers for food waste, helps minimize fly attractants. Additionally, composting should be done in a way that reduces fly access. -
Manure Management:
Livestock farms are prime breeding grounds for houseflies due to the abundance of manure. Practices like regularly turning manure piles, storing manure in covered containers, or using waste treatments to reduce fly larvae can reduce fly populations. -
Improving Drainage:
Eliminating standing water and improving drainage in agricultural fields and residential areas helps reduce fly breeding sites. Flies often breed in moist environments like puddles, ditches, and gutters. -
Use of Fly-Repellent Plants:
Certain plants, such as basil, lavender, and eucalyptus, are natural repellents to flies. Cultivating these plants around homes or farms can help keep flies away.
By modifying the environment to limit the resources that houseflies need for reproduction, cultural control methods can be highly effective.
c) Chemical control of housefly
Answer:
c) Chemical Control of Houseflies
Chemical control involves the use of insecticides to kill or repel houseflies. While it is effective in reducing fly populations, chemical control must be used cautiously to avoid resistance development and harm to non-target species.
-
Insecticide Sprays:
Aerosol sprays are widely used in homes, restaurants, and food-processing areas to quickly kill adult houseflies. Common active ingredients in these sprays include pyrethroids like permethrin and deltamethrin, which are neurotoxic to insects. -
Baited Insecticides:
Chemical fly baits are used to attract and poison flies. The bait typically contains an insecticide mixed with an attractant, which the flies ingest and then die. These are often used for larger fly infestations in confined spaces. -
Larvicides:
Larvicides are chemicals designed to kill fly larvae before they become adults. These can be applied to manure piles, compost heaps, or trash bins where flies breed. Examples of larvicides include organophosphates or insect growth regulators (IGRs) like methoprene, which inhibit larval development. -
Space Sprays:
These sprays release insecticide droplets into the air to kill flying adults. Space sprays are commonly used in areas with high fly populations, such as kitchens and warehouses.
While chemical control can provide immediate relief, it is typically recommended to integrate it with other control methods to prevent resistance buildup in fly populations.
d) Myiasis
Answer:
d) Myiasis
Myiasis refers to the infection or infestation of tissues or organs in humans or animals by the larvae (maggots) of flies. It is a condition most commonly caused by various species of Diptera, especially Calliphoridae (blowflies) and Sarcophagidae (flesh flies), although other flies like Musca domestica can also cause myiasis in certain situations.
Types of Myiasis:
-
Cutaneous Myiasis:
The larvae infest the skin, causing lesions that can range from mild irritation to severe ulceration. This type of myiasis is often seen in wounds, particularly in neglected or unsanitary conditions. -
Cavitary Myiasis:
This type affects body cavities such as the mouth, nose, ears, or eyes. The larvae may enter these cavities to feed on tissues and fluids. -
Intestinal Myiasis:
In rare cases, larvae may be ingested and invade the gastrointestinal tract, leading to symptoms like abdominal pain, nausea, and vomiting. This can occur when contaminated food is consumed. -
Urogenital Myiasis:
This occurs when fly larvae infest the urogenital tract, often in people with poor hygiene or in those who are bedridden.
Symptoms of Myiasis:
- Painful swelling at the site of infestation
- Presence of maggots in lesions or wounds
- Discharge or fluid leakage from affected areas
- In severe cases, systemic infection, fever, and shock can occur if left untreated
Treatment:
- Manual Removal: Maggots can be removed surgically or manually, often with the help of forceps.
- Topical Treatments: Antiseptic creams or ointments may be applied to prevent secondary bacterial infection after larvae removal.
- Antibiotics: Infections caused by secondary bacteria may require antibiotic therapy.
In most cases, myiasis can be prevented through good hygiene practices, prompt treatment of wounds, and effective fly control measures in areas prone to fly infestation.
Question:-10
a) Explain the concept of Integrated Vector Management.
Answer:
1. Introduction to Integrated Vector Management (IVM)
Integrated Vector Management (IVM) is a comprehensive, sustainable, and cost-effective strategy designed to control vector-borne diseases. Vectors, such as mosquitoes, ticks, and flies, transmit diseases like malaria, dengue, Zika, and Lyme disease. IVM combines different vector control measures and integrates them with environmental, social, and health interventions. The ultimate goal of IVM is to reduce the burden of vector-borne diseases, minimize the use of chemical pesticides, and enhance the resilience of communities to these diseases. IVM takes a multifaceted approach that considers both the biology of the vector and the socio-economic factors that affect disease transmission.
2. Components of IVM
IVM integrates multiple components to manage vector populations efficiently:
-
Biological Control:
This involves the use of natural predators, pathogens, or competitors to control vector populations. For example, introducing fish that feed on mosquito larvae in breeding sites or using bacterial toxins like Bacillus thuringiensis to target mosquito larvae. These methods are environmentally friendly and reduce reliance on chemical control. -
Chemical Control:
While IVM minimizes chemical control, it still employs insecticides when necessary. However, the emphasis is on targeted, judicious use to prevent the development of insecticide resistance. IVM encourages the use of the most appropriate insecticides and their rotation to avoid resistance. -
Environmental Management:
Environmental management focuses on modifying or eliminating the habitats where vectors breed. For example, draining stagnant water, covering water containers, and removing waste materials that provide breeding grounds for mosquitoes. Proper urban planning and waste management are crucial to reducing breeding sites. -
Legislation and Policy:
Effective policies and legislation are essential for the success of IVM. These may include regulations on the use of insecticides, the management of public spaces, or measures for the surveillance of vector populations. Policies must be evidence-based and adapted to local conditions. -
Community Involvement and Education:
Community participation is a cornerstone of IVM. Educating the public about vector control methods, personal protection (e.g., using insect repellent, bed nets), and the importance of maintaining a clean environment is vital for the long-term success of vector control programs. Community-based interventions empower individuals and households to take control of their own health and environment.
3. Principles of Integrated Vector Management
The concept of IVM is guided by several key principles that ensure a holistic and effective approach to vector control:
-
Sustainability:
IVM seeks to implement long-term solutions for vector control, rather than relying on short-term fixes. Sustainable strategies ensure that vector populations are controlled without harming the environment or causing economic burdens. Sustainability also involves building local capacity for ongoing management and surveillance. -
Flexibility:
The needs of vector control programs vary depending on the specific geographic region, disease, and vector species involved. IVM allows for flexibility in choosing the most appropriate methods for a given situation, whether it’s through biological control in one area or chemical control in another. -
Evidence-based Decision Making:
IVM relies on robust data and scientific evidence to make informed decisions. Surveillance and monitoring of vector populations, disease incidence, and the effectiveness of control measures are essential for adjusting interventions. This principle ensures that the strategies used are based on current understanding and best practices. -
Community-centered Approach:
The success of IVM is closely linked to community participation. Engaging the community in the planning and execution of vector control efforts ensures that interventions are culturally acceptable, practical, and more likely to be sustained. It fosters local ownership and responsibility for vector control activities. -
Multidisciplinary Collaboration:
IVM encourages the involvement of various sectors, such as public health, environmental science, urban planning, and education. Collaboration between different stakeholders, including governments, NGOs, and local communities, is key to addressing the complex nature of vector-borne diseases.
4. Benefits of Integrated Vector Management
-
Cost-effectiveness:
By combining multiple control strategies, IVM optimizes resource use and reduces the overall cost of vector control. For instance, the use of biological control methods can decrease dependence on expensive chemical pesticides. -
Reduction in Resistance Development:
Over-reliance on chemical pesticides often leads to resistance in vector populations, making control efforts less effective over time. IVM reduces this risk by incorporating non-chemical strategies and rotating insecticides, which helps to slow down the development of resistance. -
Environmental Protection:
IVM prioritizes environmentally friendly methods, such as biological control, which reduces the negative impacts on ecosystems. Reducing pesticide use also helps protect non-target species, including beneficial insects like pollinators. -
Improved Health Outcomes:
By reducing the vector population and minimizing the spread of diseases, IVM directly contributes to better public health outcomes. Fewer cases of diseases like malaria, dengue, and Zika lead to reduced morbidity and mortality, improving the overall quality of life.
5. Challenges in Implementing IVM
While IVM offers numerous benefits, its implementation can be challenging due to several factors:
-
Resource Constraints:
In many regions, particularly in low-income countries, there may be insufficient resources for comprehensive IVM programs. Lack of funding, infrastructure, and trained personnel can hinder the successful implementation of integrated approaches. -
Coordination and Collaboration:
Effective IVM requires the coordination of multiple stakeholders across various sectors. Achieving this level of collaboration can be difficult, especially when stakeholders have different priorities or lack experience working together. -
Community Acceptance:
While community participation is a key principle, ensuring that the local population is willing to adopt new behaviors and control measures can be challenging. Cultural beliefs, lack of awareness, and resistance to change may limit the effectiveness of community-based approaches. -
Surveillance and Monitoring:
Continuous surveillance and monitoring are essential for the success of IVM, but setting up and maintaining these systems can be logistically challenging and resource-intensive. Effective data collection, analysis, and sharing are crucial for making informed decisions and adapting strategies.
Conclusion
Integrated Vector Management represents a comprehensive, sustainable approach to controlling vector-borne diseases. By integrating biological, chemical, environmental, and educational strategies, IVM seeks to reduce vector populations, prevent the spread of diseases, and protect both human health and the environment. Although the implementation of IVM can be challenging, its benefits, such as cost-effectiveness, environmental sustainability, and reduction of resistance, make it a valuable strategy for managing vector-borne diseases worldwide. Success in IVM depends on careful planning, multidisciplinary collaboration, and strong community involvement to create long-lasting impact.
b) How can the disease vectors be genetically manipulated to reduce their population?
Answer:
1. Introduction to Genetic Manipulation of Disease Vectors
Genetic manipulation of disease vectors refers to the use of advanced biotechnological techniques to alter the genetic makeup of organisms that transmit diseases. The goal of this manipulation is to reduce the population of these vectors or even make them incapable of transmitting the diseases they carry. This approach offers a promising alternative or complement to traditional vector control methods such as insecticides and environmental management. In recent years, various strategies, including gene drive technologies and genetic engineering, have been explored to target the vectors of diseases like malaria, dengue, and Zika, which are transmitted primarily by mosquitoes, such as Anopheles and Aedes species.
2. Gene Drive Technology
Gene drive is a powerful genetic manipulation tool that increases the probability of a specific gene being passed down to the offspring, spreading it rapidly through a population. Unlike traditional Mendelian inheritance, where a gene has a 50% chance of being inherited, a gene drive forces nearly 100% inheritance of the gene in question.
-
How Gene Drive Works:
Gene drive systems are typically built using CRISPR/Cas9, a gene-editing technology. In this system, a genetic modification is designed to spread through the population by ensuring that the modification is passed on not just from one parent to offspring but also alters the other parent’s gene to carry the same modification. This allows the gene to propagate through the entire population at a faster rate than it would under normal inheritance patterns. -
Applications for Disease Vectors:
Gene drives can be used to engineer mosquitoes in various ways:- Sterility: Gene drive systems can be used to cause infertility in mosquitoes, reducing the population by preventing the production of viable offspring.
- Resistance to Pathogens: Another strategy involves creating genetically modified mosquitoes that are resistant to the pathogens they carry, such as Plasmodium (which causes malaria) or the dengue virus. This prevents the mosquitoes from transmitting the diseases.
- Population Suppression: By introducing a gene drive that causes mosquitoes to develop as males only (a technique known as the "X-shredder"), it is possible to skew the sex ratio and reduce the overall population.
Gene drive offers a potentially transformative solution for vector control, but it raises ethical and ecological concerns, including the risks of unintended consequences on ecosystems and the spread of genetically modified organisms (GMOs) beyond the target areas.
3. Release of Sterile Insects
The sterile insect technique (SIT) involves releasing large numbers of genetically sterilized male mosquitoes into the wild. These males mate with females in the wild, but since they are sterile, no offspring are produced. Over time, this reduces the population of mosquitoes.
-
How Sterility is Achieved:
Sterility can be induced in insects through radiation or genetic modification. In the genetic modification approach, males can be engineered to carry a gene that causes infertility. This method has been successfully applied in mosquito populations, particularly in the context of controlling diseases like malaria and dengue. -
Advantages and Challenges:
One of the primary advantages of SIT is that it does not involve the use of chemical insecticides, making it environmentally friendly. However, the technique requires the mass production and release of sterile males, which can be expensive and logistically challenging. Additionally, for SIT to be effective, the mosquitoes must be able to compete with wild males for mates, which may not always be the case.
4. Population Replacement Strategies
Population replacement involves the introduction of genetically modified mosquitoes into the wild population that are incapable of transmitting pathogens. Unlike population suppression, which reduces the number of mosquitoes, population replacement focuses on making the surviving mosquitoes less harmful to humans.
-
Genetic Modification to Prevent Pathogen Transmission:
One approach to population replacement involves genetically modifying mosquitoes to be resistant to pathogens like Plasmodium or the dengue virus. By introducing such mosquitoes into the wild population, the overall ability of the mosquito population to transmit disease is reduced. For example, some mosquitoes have been genetically altered to carry a gene that makes them resistant to the malaria parasite, thus preventing the parasite from developing within the mosquito and being transmitted to humans. -
Wolbachia Bacteria:
Another innovative approach involves infecting mosquitoes with Wolbachia, a type of bacteria that sterilizes mosquitoes or prevents them from being able to transmit pathogens. Wolbachia infection has been successfully used in mosquitoes to prevent the transmission of viruses like dengue and Zika. This method involves releasing mosquitoes infected with Wolbachia into the wild population, where they spread the bacteria, reducing the population’s ability to transmit diseases over time.
5. CRISPR/Cas9 and Gene Editing Technologies
CRISPR/Cas9 technology has revolutionized genetic engineering by allowing scientists to precisely edit genes within an organism. This has opened up new avenues for the manipulation of disease vectors, particularly mosquitoes.
-
Editing the Mosquito Genome:
CRISPR/Cas9 can be used to target specific genes in mosquitoes that influence traits like pathogen susceptibility or reproduction. For example, scientists can alter genes related to the mosquito’s immune system to make it resistant to diseases like malaria. Alternatively, CRISPR/Cas9 can be used to induce genetic changes that render mosquitoes sterile or cause them to produce offspring that die early in development. -
Challenges and Ethical Concerns:
While CRISPR/Cas9 offers precise control over genetic changes, its application to disease vectors comes with challenges. One concern is the potential for off-target effects, where unintended genes might be edited, leading to unintended consequences. Moreover, the release of genetically modified mosquitoes into the wild raises ethical issues, such as the potential impact on biodiversity and the ecosystem.
6. Ecological and Ethical Considerations
While genetic manipulation offers powerful tools for controlling disease vectors, it raises several ecological and ethical concerns:
-
Impact on Ecosystems:
The introduction of genetically modified organisms into natural ecosystems could have unforeseen consequences. For instance, altering mosquito populations may affect the species that rely on mosquitoes for food, such as birds and bats. Additionally, genetic modifications that reduce mosquito populations might have cascading effects on the ecosystems they inhabit. -
Ethical Dilemmas:
The use of gene drives and genetically modified mosquitoes introduces ethical debates around human intervention in natural processes. Concerns include the potential for irreversible changes in the environment, the possibility of releasing genetically altered organisms without fully understanding the long-term impacts, and the rights of communities who might be affected by such interventions.
Conclusion
Genetic manipulation of disease vectors offers a revolutionary approach to controlling vector-borne diseases by directly targeting the organisms responsible for transmission. Techniques like gene drive, sterile insect release, and genetic modification hold promise for reducing the population of disease vectors, preventing pathogen transmission, and even eliminating certain diseases. However, these technologies must be approached with caution, given the potential ecological and ethical risks. As research and technology advance, the integration of genetic manipulation with other vector control methods will be essential for the sustainable management of vector-borne diseases.